Introduction
Corrosion losses in India are estimated at about 6500 US dollars per year 1. At high temperatures, corrosion can lead to hot corrosion, a process that accelerates oxidation 2. This occurs when the metal surface is covered with a salt layer. Numerous reactive species, such as ash content, moisture content, and alkali earth metal content, are produced as a result of fuel combustion, and these substances are highly corrosive in nature. Potassium, chlorine, sulfur, calcium, and silicon salts are formed as a result of rice husk combustion. 3. Deposits of these salt species can accumulate on the surfaces of fireside components. The working temperature in boiler environments ranges from 500°C to 900°C 4-5. When boiler components are subjected to high temperatures, a layer of oxide scale develops on their surfaces as a protective measure. The combination of salt species with the protective oxide layer leads to the dissolution of the oxide, leaving the component vulnerable to corrosion. The chlorine present in the environment influences the protective oxides by forming the gas phases of Cl2, HCl, NaCl, and KCl. When the salt species dissolve the protective oxide layer, the surface becomes vulnerable to active oxidation, which can increase the rate of oxidation and lead to direct corrosion. 6,7. Corrosion in boilers under a high-temperature environment is known as hot corrosion. This is induced by a thin film of fused salt deposits present in the environment. The two main types of hot corrosion are Type 1, also known as high-temperature hot corrosion, and Type 2, also known as low-temperature hot corrosion. Significant economic losses can be incurred as a result of hot corrosion, which may necessitate extra maintenance or forced outages of boiler materials 8.
The considerable efforts have been done to reduce the economic losses caused due to corrosion attack. To mitigate the effects of hot corrosion, different strategies have been implemented, including incorporating corrosion inhibitors, using high-chromium materials, and applying anti-corrosion coatings. Out of all the available methods, the application of coatings is considered to be the most efficient and effective approach as the use of inhibitors has proven to be inadequately successful, and incorporating materials with high chromium content would significantly escalate the expenses 9. The coating materials used in high temperature applications must have the characteristics to form a stable and the slow-growing surface oxide in order to provide good service behaviour 10. Various coating processes are used to improve the surface properties of various metals for different applications. The thermal spray coating process is a widely recognized method for enhancing surface wear resistance, with variations such as plasma spray, arc spray, detonation gun spray, and flame spray 11.
This paper aimed to present the different coating deposition techniques, coatings, and their behaviour in high temperature conditions of boiler environments.
Types of hot corrosion
There are two types of hot corrosion that can occur at different temperature ranges. Low temperature hot corrosion, also known as Type II, happens at temperatures between 600-750°C, while high temperature hot corrosion, also known as Type I, occurs at temperatures between 800-950°C. 8 Various factors, such as velocity, alloy composition, temperature cycles, and erosion process can impact both types of hot corrosion. Type I hot corrosion is caused by a fluxing process where the chemistry of sodium sulfate deposit is altered, allowing sulfur to penetrate the metal underneath. This penetration causes a reduction in the chromium content of the substrate metal, leading to oxidation and the formation of a porous oxide scale. In Type-II hot corrosion, sulfates of the base metal react with alkali metal, resulting in the creation of a eutectic with a low melting point that inhibits the development of protective oxides 12. Due to their lower melting point, Ni-based coatings are more prone to degradation in environments containing sulfur under Type-II hot corrosion. Type-II corrosion is characterized by pitting attacks and, under microscopic observation, shows minimal sulfide formation with no indications of chromium depletion 13.
Mechanism of Hot corrosion
High-temperature hot corrosion starts with the breakdown of the protective oxide layer, which then allows the molten salt to directly attack the underlying substrate material. This breakdown can result from erosion, erosion-corrosion, thermal stresses, and chemical reactions. Two mechanisms have been proposed for the propagation stages of Type-I hot corrosion, namely sulphidation-oxidation and salt fluxing. 14. Figure 1 shows the schematic illustration of reactive/corrosive environment surrounds the biomass fired boiler. At first, the salt fluxing mechanism was suggested by Goebel and Pettit et al., which implies that the molten salt may cause the surface oxide layer to lose its protective efficiency due to fluxing of this layer. This can be either basic type or acidic type of fluxing. Basic fluxing occurs when oxides combine with oxygen to form anions, whereas acidic fluxing occurs when oxides decompose into cations and oxygen. Acidic fluxing is more severe than basic fluxing because it leads to a more intense oxidation reaction, especially when the oxidation activity in the molten salt is significantly reduced. On the contrary to basic fluxing, acidic fluxing has the ability to sustain itself, as the degree of salt displacement from stoichiometry does not intensify with the advancement of the reaction 15.
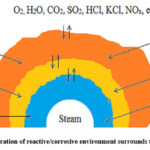 |
Figure 1: Schematic illustration of reactive/corrosive environment surrounds the biomass fired boiler 16
Click here to View Figure
|
Various stages in Hot Corrosion
The hot corrosion mechanism of boiler steels typically involves several stages leading up to component failure:
Stage I: (Incubation Period) In the beginning phase, the reaction follows a pattern comparable to typical oxidation reactions.
Stage II: (Initiation Stage) At this point, corrosion is accelerated compared to the incubation stage.
Stage III: (Propagation Stage) During this stage, corrosion occurs at a very rapid rate.
After Stage III, the components ultimately fail 16.
Thermal Spray Coatings
Thermal spray coatings encompass various techniques aimed at managing the detrimental effects of high temperatures. This approach involves applying an additional layer of materials that are resistant to degradation onto the surface of steel. This layer serves to impede the rate of corrosion-oxidation, thereby slowing down or preventing material degradation, which can result in a prolonged operational lifespan for the component. The process of thermal spray coating entails the application of safeguarding substances, like ceramics, metals, or specific polymeric materials, onto the surface of the underlying substrate that necessitates protection. 17,18. The spray gun receives the coating material and expels it onto the substrate surface at a high velocity after heating it. The material adheres to the substrate surface through a combination of adhesion and diffusion upon solidification. Flame spray, electric arc deposition, plasma spray coating, high velocity oxy-fuel coating, detonation gun deposition, and cold spray coating are among the thermal spray processes that exist. Each of these methods has its advantages and disadvantages. To select the appropriate thermal spray process, the bond strength, spray velocity, oxide formation, etc. factors among others are taken into consideration. The suitability of each available technique is determined by these factors. 19.
Coatings tested under aggressive environments by various researchers
The comprehensive review of recent research papers focussing on the studies about the high temperature corrosion behaviour of different coatings in the reactive environments is presented in Table 1 as follows:
Table 1: Tabular representation of various behavioural studies of coatings under aggressive environments.
S. No.
|
Author
|
Substrate
|
Coating
|
Coating Method
|
Environment
|
1
|
Reddy et al., 20
|
MDN 420 alloy
|
70% NiCrAlY+ 25% Cr2O3+5% YSZ and 70% NiCrAlY+ 30% TiO2
|
Plasma spray method
|
Mixture salt of Na2SO4+60 % V2O5 at 700°C
|
2
|
Dolekar et al., 21
|
Inconel 718
|
CoNiCrAlY + YSZ and YSZ/Gd2Zr2O7
|
EB-PVD
|
Mixture of salts of NaCl, Na2SO4, and V2O5 at 1000 °C
|
3
|
Singh et al., 22
|
T22
|
Ni-22Cr-10Al-1Y- SiC (N) and Ni22Cr-10Al-1Y
|
HVOF
|
At 900°C in Na2SO4-60wt%V2O5 molten-salt environment.
|
4
|
Singh et al., 23
|
T22
|
NiCrAlY-SiC and NiCrAlY-B4C
|
HVOF
|
Na2SO4+60% V2O5 environment at high temperature of 900° C
|
5
|
Singh et al., 24
|
T22
|
Ni-22Cr-10Al-1Y-B4C(nano sized) and Ni-22Cr-10Al-1Y-B4C (micro sized)
|
HVOF
|
In SiC tube furnace at temperature of 900°C
|
6
|
Singh et al., 25
|
T91
|
NiCrAlY
|
HVOF
|
At a temperature of 900°C in normal air SiC tube furnace
|
7
|
Lone et al., 26
|
Superni-75
|
Cerium oxide
|
Electroless process
|
In molten salt environment of Na2SO4–60%V2O5 at 900 °C
|
8
|
Bala et al., 27
|
SA 516 alloys and SA-213-T22
|
Ni-50Cr
|
Cold spray process
|
Na2SO4–60% V2O5 under cyclic conditions and at high temperature of 900 °C.
|
9
|
Mittal et al., 28
|
T91
|
Ni-Cr and Stellite-21
|
D-Gun spray process
|
In the presence of air and Na2SO4-60% V2O5 salt mixture at 900˚C
|
10
|
Nithin et al., 29
|
MDN 321 and Superni 76
|
CoCrAlY + Al2O3 + YSZ and CoCrAlY + CeO2 and NiCrAlY as a bond coat
|
Plasma sprayed
|
In Na2SO4-60%V2O5 enviroment exposed to 700 °C
|
11
|
Dolekar et al., 30
|
Inconel 718
|
CoNiCrAlYas bond and yttria-stabilized zirconia (YSZ) and YSZ/Gd2Zr2O7
|
HVOF and Electron beam physical vapor deposition (EB-PVD)
|
30% NaCl, 35% V2O5, and 35% Na2SO4 at 1000 °C
|
12
|
Singh et al., 31
|
T-91
|
Ni3Al and TiO2
|
HVOF
|
Na2SO4-60% V2O5 environment at 900ºC
|
13
|
Singh et al., 32
|
T22
|
Ni–20Cr, Ni–20Cr–TiC and Ni– 20Cr–TiC–Re
|
Cold spray process
|
Na2SO4-60%V2O5 at 900ºC
|
14
|
Jafari and Sadeghi33
|
16Mo3 and Sanicro-25 steels
|
Ni21Cr, Ni5Al, and Ni21Cr7AlY
|
HVAF
|
At 600 °C for 168 h in ambient air under KCl and 50-50 mol% KCl–K2SO4 salts
|
15
|
Sadeghimeresht et al., 34
|
16Mo3
|
NiCrAlY and NiCrMo coatings
|
HVAF
|
Chloridizing-oxidizing environment with and without a KCl deposit, at 600 °C
|
16
|
Eklund et al., 35
|
16Mo3
|
NiCr, NiAl and NiCrAlY
|
HVAF
|
O2 + H2O and O2 + H2O + KCl at 600°C
|
17
|
Sundaresan et al., 36
|
T91
|
CoCrAlY, NiCoCrAlY, and NiCr
|
Atmospheric Plasma Spray (APS) and Detonation spray (DSC)
|
Na2SO4-K2SO4-Fe2O at 650°C
|
18
|
Singh et al., 37
|
T22
|
65NiCr–Cr3C2, 80NiCr–Cr3C2, 90NiCr–Cr2C3 and NiCr
|
HVOF
|
Na2SO4-60 wt% V2O5 salts at 800°C
|
19
|
Singh et al., 38
|
T22
|
100wt%Cr2O3, 90wt% Cr2O3–10wt%TiO2, and 80wt%Cr2O3–20wt%TiO2
|
HVOF
|
Na2SO4–60wt%V2O5
|
20
|
Wang et al., 39
|
T22
|
NiCrB and NiCrTi
|
Arc sprayed
|
Na2SO4-10 wt.% NaCl salt at temperature of 800 °C
|
21
|
Abu-warada et al., 40
|
T24 and T92 steels
|
NiMoCrW and CoNiCrAlY
|
HVOF
|
Nabertherm LT 5/12/P330 furnace by following the standard ISO/FDIS 17224:2014 at 650 ◦C
|
22
|
Bhatia et al., 41
|
T91
|
75% Cr3C2– 25% (Ni-20Cr)
|
HVOF
|
Na2SO4-60 wt.% V2O5 at 550,700 and 850°C.
|
23
|
Singh et al.,42
|
T22
|
Cr2O3
|
Plasma sprayed
|
60% NaSO4–40% V2O5 at 850°C
|
24
|
Zhou et al., 43
|
P91
|
Cr3C2-NiCrMoNbAl and Cr3C2-NiCr
|
HVOF
|
6.5% NaCl+34.5% KCl+5 % Na2SO4 at temperature of 650°C
|
25
|
Bala et al., 44
|
SA516
|
Ni-50Cr and Ni-20Cr
|
HVOF
|
At 700 ± 10 °C and volumetric flow of flue gases 16% CO2 and 3% O2
|
26
|
Sadeghi and Joshi 45
|
EN 16Mo3
|
FeCrNiMoBSiC coatings
|
HVOF and HVAF
|
At temperature of 600 ± 3 °C with and without KCl salt
|
27
|
Jafari et al., 46
|
16Mo3
|
Ni21Cr, Ni5Al, Ni21Cr7Al1Y and Ni21Cr9Mo coatings
|
HVAF
|
at temperature 600°C in an air furnace up to 168 h in presence of KCl
|
28
|
Fantozzi et al., 47
|
P92
|
Cr3C2-25NiCr, Cr3C2-50NiCrMoNb and Cr3C2-37WC18NiCoCr coatings
|
HVOF and HVAF
|
At temperature of 400, 450, 500 and 550 °C under KCl deposits
|
29
|
Kaushal et al., 48
|
T22
|
Ni20Cr
|
HVOF , D-Gun, and cold sprayed
|
Na2SO4-60% V2O5 at 1173 K (900 °C)
|
30
|
Kai et al., 49
|
ASTM1020 steel
|
NiCrC conventional and nano coating
|
HVOF
|
At temperature of 500-750°C under Na2SO4–30% K2SO4
|
31
|
Liu et al., 50
|
TP347H stainless steel, laser-cladded C22 and C22 alloy
|
–
|
–
|
In molten alkali chloride salts at temperature of 450–750°C
|
32
|
Zhen et al., 51
|
Inconel-740
|
–
|
–
|
In simulated conditions at temperature of 750°C
|
33
|
Awadi et al., 52
|
Inconel 738 and 617
|
–
|
–
|
At temperature ranges of 700, 800 and 900°C in aggressive environment of NaCl and Na2SO4
|
34
|
Rani et al., 53
|
ASTM-SA210-A1
|
Cr2O3-75%Al2O3
|
Detonation gun spray technique
|
Na2SO4-60%V2O5 at 900°C
|
35
|
Thakare et al., 54
|
P91
|
75Cr3C2-25NiCr
|
Detonation gun
|
boiler environment at 650°C
|
36
|
Somervuori et al., 55
|
AISI 316 steel plates
|
NiCr and NiCrBSi
|
APS, HVOF, and HVAF.
|
0.5% and 3.5% NaCl solutions
|
37
|
Abu-warda et al., 56
|
T24
|
NiCr
|
HVOF
|
KCl, NaCl, Na2SO4 and K2SO4 at 650 ºC
|
38
|
Matikainen et al.,57
|
Metal substrates
|
Cr3C2-50NiCrMoNb and Cr3C2– 37WC-18NiCoCrFe
|
HVAF and HVOF
|
–
|
39
|
Chatha et al., 58
|
T91
|
Cr3C2-NiCr
|
HVOF
|
Na2SO4–60% V2O5 at 900 °C
|
40
|
Mudgal et al., 59
|
Superni 718
|
Cr3C2-NiCr + 0.2wt.%Zr and Cr3C2-NiCr
|
D-Gun spray process
|
40%Na2SO4 + 10%NaCl + 40%K2SO4 + 10%KCl. and 75%Na2SO4 + 25%NaCl.
|
41
|
Mudgal et al., 60
|
Superni 600
|
Cr3C2-25(NiCr) +0.4 %CeO2 and Cr3C2-25(NiCr)
|
D-gun technique
|
Na2SO4-25%NaCl
|
42
|
Mudgal et al.,61
|
Superni 600, Superni 718, and Superni 605
|
–
|
–
|
Na2SO4–25%NaCl) at 900 °C
|
43
|
Ahuja et al., 62
|
Superni 718, Superni 600 and Superco 605
|
Cr3C2-(NiCr)-0.2 wt% of Zr
|
D-gun technique
|
40%Na2SO4-40%K2SO4-10%NaCl-10%KCl at 900 °C
|
44
|
Goyal et al., 63
|
T22
|
CNT+Cr3C2–20NiCr
|
HVOF technique
|
In zone of super-heater zone in actual environment of boiler of a thermal power plant at 900°C
|
45
|
Sidhu et al., 64
|
T22
|
93(WCCr3C2)-7Ni and 83WC-17Co coatings
|
HVOF technique
|
In coal fired boiler environment of 900°C in superheater zone.
|
46
|
Sidhu et al.,65
|
T91
|
83WC-17Co, 86WC-10Co-4Cr, 93(WC-Cr3C2)-7Ni, 75Cr3C2-25NiCr,
|
HVOF Technique
|
In actual boiler environment at an elevated temperature of 900◦C in superheater
|
47
|
Goyal et al., 66
|
ASTM-SA213-T22
|
Cr2O3–CNT
|
HVOF technique
|
In a molten salt environment of Na2SO4+60wt%V2O5 salt at 700°C.
|
48
|
Goyal et al., 67
|
ASTM-SA213-T22
|
Cr2O3 + 1, 2, 4, 6, and 8 wt-% of CNT
|
HVOF Technicque
|
In real power plant boiler environment at high temperature of 900°C.
|
The following outcomes were discussed in these studies:
Reddy et al., 20 stated that Cr2O3 has less solubility in highly acidic molten salt mixture of Na2SO4-60%V2O5. According to their study, the presence of chromium oxide formed around the Cobalt and Nickel-rich splats present in the pores could have acted as barriers against the penetration and diffusion of corrosive species, blocking their passages. Dolekar et al., 21 demonstrated that, in comparison to the conventional YSZ TBC system, the YSZ/Gd2Zr2O7 layer demonstrated better performance in terms of hot corrosion and oxidation. This was due to the pre-sacrificial layer of Gd2Zr2O7 in YSZ/Gd2Zr2O7 content, which lessen the damage to the YSZ layer. Singh et al., 22 in their paper, defined that the subsurface inter-splats in the composite coating of Ni-22Cr-10Al-1Y-SiC (N) deposited on T22 by HVOF process exhibited favourable results against hot corrosion due to the presence of SiO2. In another study, Singh et al., 23 arrived at the conclusion of the formation of silicon dioxide accompanied by oxides of Nickle, Aluminium, and Chromium which have proved to be more resistive to corrosion than the formation of B2O3 with these oxides. Singh et al., 24,25 concluded that the use of nano coatings provided greater protection compared to micro coatings. This is due to the formation of oxides of boron resulting from the full melting of nano particles of boron carbide within the inner splats, in combination with oxides of chromium, nickel, aluminum, and a nickel/chromium spinel. Lone et al., 26 revealed that cerium concentration in the cerium oxide-coated Superni-75 samples increases when the weight variation per unit area decreases. The conclusion drawn was that the presence of a cerium oxide layer on the surface impedes the movement of ions by reducing their short circuit diffusion. Bala et al. 27 and Mittal et al., 28 found that the uncoated steels experienced severe spallation in the form of oxide scales, likely due to the formation of unprotective Fe2O3 oxide scales. In contrast, the Ni-50Cr coated steels exhibited reduced weight gains and maintained their oxide scales until the end of the experiment. The studies also revealed that the protective oxides present on the coated specimens mainly comprised of Chromium and Nickle, as well as their spinel. Nithin et al., 29 concluded that CoCrAlY + Al2O3 + YSZ (C1) has effective resistance to corrosion than CoCrAlY + CeO2 (C2) deposited on boiler steels, because of the formation of thermodynamically stable α-Al2O3 in C1 and outward growth of superficial irregular cracks CeVO4 in C2. Singh et al., 32 indicated that Ni–20Cr– TiC–Re coating offered the better corrosion resistance among different coatings of Ni–20Cr, Ni–20Cr–TiC. Jafari and Sadeghi33 and Sadeghimeresht et al., 34 found that KCl can cause significant corrosion to coatings that contain chromium, due to frequent chlorination-oxidation processes that destroy the protective chromium layer and lead to the loss of Cr through the formation of chromate. Eklund et al., 35concluded that NiAl coating exhibited excellent resistance to corrosion and effectively blocked the diffusion of all corrosive substances, including chlorine and oxygen, through the coating. Sundaresan et al.,36 concluded that detonation sprayed coatings performed better than plasma sprayed coating. In a study, Singh et al., 38 revealed that TiO2 functions as a protective barrier against corroding agents by occupying any gaps or fractures in the coating’s microstructure. Wang et al.,39 found that NiCrB coatings have greater corrosion resistance in comparison to NiCrTi coatings as a result of boron’s inclination towards producing small oxide particles, ultimately augmenting the safeguarding of oxide layers. Abu-warada et al.,40 showed that the presence of a stable Al2O3 oxide scale on the CoNiCrAlY coating reduced chloride penetration and prevented the degradation of the protective oxide scale through the formation of chromates. Bhatia et al.,41 indicated that Mo and Nb exhibited a proclivity for outward diffusion from the substrate to the coating. Zhou et al.,43 indicated that the incorporation of multiple alloying elements into the Cr3C2-NiCrMoNbAl coating led to a significant reduction in the corrosion rate compared to the Cr3C2-NiCr coating. Bala et al.,44 revealed higher microhardness of the Ni-50Cr coating than Ni-20Cr was identified as the reason behind better resistance to oxidation and erosion. Sadeghi and Joshi 45 found that the HVAF coating showed better high-temperature corrosion as compared to HVOF coating due to the formation of slightly denser protective oxides. A significant finding of the study was that the lower hardness of the HVOF coating, as compared to the HVAF coating, resulted in more substantial removal of the former during the erosion test, due to the impact of particles on the coating. Kaushal et al., 48 highlighted that the denser structure of the D-gun sprayed coating provided superior corrosion resistance in comparison to the cold spray and HVOF spray coatings. Kai et al. 49 showed that the nanostructured NiCrC coating facilitated an increased rate of grain boundary diffusion, leading to the development of a denser oxide scale with a higher growth rate. This helped to stop the depletion of chromium at the substrate/scale interface. Rani et al., 53 revealed that by utilizing the detonation gun coating technique, a uniform and strongly adherent coating with a dense microstructure was achieved. Thakare et al., 54 suggested that the creation of Cr3C2 could be attributed to the enhancement of the steel substrate’s corrosion resistance. Abu-warda et al., 56 found that the NiCr coatings tend to protect the substrate but undergo severe corrosion attacks by the chlorides. In addition to it, the formation of potassium chromate and penetration of chlorides as major causes of the attack. Matikainen et al.,57 discussed the benefits of using HVAF, such as the formation of more durable coatings with improved toughness and a more uniform coating structure. This is attributed to the higher particle velocities and temperature control achieved during the HVAF process. Chatha et al.,58 revealed that the pores in the Cr3C2–NiCr coating became blocked due to the rapid formation of oxides at the boundaries of the coating splats and within the open pores during heat treatment. This prevented corrosive substances from flowing inward towards the substrate. Mudgal et al.,59 concluded that the presence of Cr2O3 on the surface may be the reason behind corrosion resistance against the aggressive surroundings on Cr3C2-(NiCr) coating and the splat boundaries. During corrosion, the inclusion of Zr in the coating powder led to a decrease in the oxidation rate and enhanced the ability of the oxide scale to adhere to the surface of the coating. Mudgal et al.,60 conducted concluded that Cr3C2-25(NiCr)+0.4%CeO2 coating consists CeS on the surface scale and CeO2 along the splat boundaries other than Cr2O3 and spinels, The presence of CeO2 at the splat boundaries restricted the flow of reacting species which decreses the corrosion rate. Mudgal et al.,61 pointed out that the Co-based alloy experienced a greater increase in weight compared to the Ni-based alloy because the scale on the Co-based alloy was not protective in nature. Ahuja et al.,62 concluded that the substrate of Superni 600 and Superni 718 displayed the formation of a thick and dense oxide scale, whereas the corroded coated substrate of Superco 605 showed porous and loose oxide. Goyal et al.,63 noticed the lowest corrosion rate with value of 28.49 mpy was observed for Cr3C2–20NiCr -2 wt-%CNT coating on T22 steel. Sidhu et al.,64 listed the performance of coating in the order of 83WC-17Co coated T91 <83WC-17Co-coated T22 < 93(WC-Cr3C2)-7Ni coated T22 < 93(WC-Cr3C2)-7Ni-coated T91. In another study, Sidhu et al.,65 found that the presence of a thin layer of tungsten carbides, nickel oxides and chromium oxides is responsible for the coating performance on steel alloys made up of 93% WC-Cr3C2 and 7% Ni.. Goyal et al.,66, 67 found that even distribution of CNTs within the coating matrix and In addition to it, the protective nature of chromium oxides found on the surface scale exhibited superior corrosion resistance.
Conclusions
Researchers have reached the conclusion that various thermal spray coating processes can easily coat any material onto a substrate material, such as boiler steels. Among different thermal spray techniques. With its advanced and effective capabilities, the HVOF process is increasingly being used to produce high-quality coatings that exhibit high micro-hardness and low porosity. Surface modification techniques are crucial in various industries to avoid boiler failures. These techniques enhance the durability of machine parts, leading to longer lifespan and reduced replacement expenses. This results in high thickness coatings that are dense, strongly adhered to the material of substrate, and exhibit significantly greater micro-hardness as compared to the base steels. The valuable insights presented by this study can aid in selecting the optimal coating method and combination to prevent failure of boiler steels.
Conflict of Interest
There are no conflict of interest.
Funding Sources
There is no funding sources.
References
- Singh, S. K., Singh, M., Singh, A., Singh, K., Gupta, R., & Goyal, R. (2021). Performance Analysis of Computer Controlled Thermal Spraying on Hot Corrosion Control of Boiler Tube Steels. In 2021 3rd International Conference on Advances in Computing, Communication Control and Networking (ICAC3N), IEEE,1055-1060.
CrossRef - Sequeira, C. A. (2019). High temperature corrosion: fundamentals and engineering. John Wiley & Sons
CrossRef - Singh, A. K., Masto, R. E., Hazra, B., Esterle, J., & Singh, P. K. (2020). Ash from coal and Biomass combustion. Springer International Publishing.
CrossRef - Prabu, S. S., Muthu, S. M., Sujai, S., Ramkumar, K. D., Beemkumar, N., & Kariappan, E. (2023). Failure Assessment and High-Temperature Corrosion Behavior of Inconel 625 Welds in Simulated K2SO4+ 60% NaCl Boiler Environment. Journal of Materials Engineering and Performance, 1-16.
CrossRef - Sidhu, V. P. S., Goyal, K., & Goyal, R. (2017). Comparative study of corrosion behaviour of HVOF-coated boiler steel in actual boiler environment of a thermal power plant. Journal of the Australian Ceramic Society, 53, 925-932.
CrossRef - Xu, D., Guo, S., Xu, D., & Guo, S. (2022). Corrosion Types and Elemental Effects of Ni-Based and FeCrAl Alloys. Corrosion Characteristics, Mechanisms and Control Methods of Candidate Alloys in Sub-and Supercritical Water, 23-49.
CrossRef - Singh, S., Goyal, K., & Bhatia, R. (2022). A review on protection of boiler tube steels with thermal spray coatings from hot corrosion. Materials Today: Proceedings
CrossRef - Bhagria, B. K., Mudgal, D., Sidhu, S. S., & Verma, R. (2021, May). Present scenario of hot corrosion studies performed with ferritic steel. In AIP Conference Proceedings, AIP Publishing LLC, 2341, 040034.
CrossRef - Hu, S., Finklea, H., & Liu, X. (2021). A review on molten sulfate salts induced hot corrosion. Journal of Materials Science & Technology, 90, 243-254.
CrossRef - Zhang, X., Zhang, N., Xing, B., & Yin, S. (2022). An Assessment of the High-Temperature Oxidation Resistance of Selected Thermal Sprayed High Entropy Alloy Coatings. Journal of Thermal Spray Technology,31, 1-18.
CrossRef - Fauchais, P., & Vardelle, A. (2012). Thermal sprayed coatings used against corrosion and corrosive wear. Advanced plasma spray applications, 10, 34448.
CrossRef - Shifler, D. A. (2018). Hot corrosion: a modification of reactants causing degradation. Materials at High Temperatures, 35(1-3), 225-235.
CrossRef - Prashar, G., & Vasudev, H. (2020). Hot corrosion behavior of super alloys. Materials Today: Proceedings, 26, 1131-1135.
CrossRef - Eliaz, N., Shemesh, G., & Latanision, R. M. (2002). Hot corrosion in gas turbine components. Engineering failure analysis, 9(1), 31-43.
CrossRef - Hu, S., Finklea, H., & Liu, X. (2021). A review on molten sulfate salts induced hot corrosion. Journal of Materials Science & Technology, 90, 243-254.
CrossRef - Kumar, S., Kumar, M., & Handa, A. (2018). Combating hot corrosion of boiler tubes–A study. Engineering failure analysis, 94, 379-395.
CrossRef - Kong, D., Dong, C., Ni, X., & Li, X. (2019). Corrosion of metallic materials fabricated by selective laser melting. npj Materials Degradation, 3(1), 24.
CrossRef - Goyal, K., Singh, H., & Bhatia, R. (2016). Current status of thermal spray coatings for high temperature corrosion resistance of boiler steel. Journal of Material & Metallurgical Engineering, 6(1), 29-35.
- Prashar, G., Vasudev, H., & Thakur, L. (2021). Thermal Spraying Fundamentals: Process Applications, Challenges, and Future Market. Thermal Spray Coatings, 1-36.
CrossRef - Reddy, M., Prasad, C. D., Patil, P., Ramesh, M. R., & Rao, N. (2021). Hot corrosion behavior of plasma-sprayed NiCrAlY/TiO2 and NiCrAlY/Cr2O3/YSZ cermets coatings on alloy steel. Surfaces and Interfaces, 22, 1-11.
CrossRef - Doleker, K. M., Ozgurluk, Y., Kahraman, Y., & Karaoglanli, A. C. (2021). Oxidation and hot corrosion resistance of HVOF/EB-PVD thermal barrier coating system. Surface and Coatings Technology, 409, 1-13.
CrossRef - Singh, G., Bala, N., & Chawla, V. (2020). Microstructural analysis and hot corrosion behavior of HVOF-sprayed Ni-22Cr-10Al-1Y and Ni-22Cr-10Al-1Y-SiC (N) coatings on ASTM-SA213-T22 steel. International Journal of Minerals, Metallurgy and Materials, 27(3), 401-416.
CrossRef - Singh, G., Bala, N., Chawla, V., & Singla, Y. K. (2021). Hot corrosion behavior of HVOF-sprayed carbide based composite coatings for boiler steel in Na2SO4–60% V2O5 environment at 900° C under cyclic conditions. Corrosion Science, 190, 1-13.
CrossRef - Singh, G., Bala, N., & Chawla, V. (2019). High Temperature oxidation behaviour and characterization of NiCrAlY-B4C coatings deposited by HVOF. Materials Research Express, 6(8), 1-15.
CrossRef - Singh, G., Bala, N., & Chawla, V. (2017). High temperature oxidation behaviour of HVOF thermally sprayed NiCrAlY Coating on T-91 boiler tube steel. Materials Today: Proceedings, 4(4), 5259-5265.
CrossRef - Lone, S. A., & Rahman, A. (2020). Hot Corrosion Behaviour of Electroless Deposited Nano-structured Cerium Oxide Coatings on Superalloy. Journal of The Institution of Engineers (India): Series D, 101(1), 81-92.
CrossRef - Bala N, Singh H and Prakash S (2010). Accelerated hot corrosion studies of cold spray Ni–50Cr coating on boiler steels, Mater. Des., 31, 244–53
CrossRef - Mittal, R., Singh, M., & Kumar, P. (2019). Characterization of detonation gun thermal spray coatings on SA213T91 in simulated boiler environment. Materials Today: Proceedings, 18, 4952-4962.
CrossRef - Nithin, H. S., Desai, V., & Ramesh, M. R. (2018). Hot corrosion behaviour of refractory and rare earth oxide reinforced CoCrAlY APS coatings at 700° C. Transactions of the Indian Institute of Metals, 71(10), 2403-2413.
CrossRef - Doleker, K. M., Ozgurluk, Y., Kahraman, Y., & Karaoglanli, A. C. (2021). Oxidation and hot corrosion resistance of HVOF/EB-PVD thermal barrier coating system. Surface and Coatings Technology, 409, 1-13.
CrossRef - Singh, S., Goyal, K., & Goyal, R. (2016). Performance of Ni3Al And TIO2 coatings on T22 boiler tube steel in simulated boiler environment in laboratory. Journal of Mechanical Engineering, 46(1), 54-61.
CrossRef - Singh, H., Bala, N., Kaur, N., Sharma, S. K., Kim, D. Y., & Prakash, S. (2015). Effect of additions of TiC and Re on high temperature corrosion performance of cold sprayed Ni–20Cr coatings. Surface and Coatings Technology, 280, 50-63.
CrossRef - Jafari, R., & Sadeghi, E. (2019). High-temperature corrosion performance of HVAF-sprayed NiCr, NiAl, and NiCrAlY coatings with alkali sulfate/chloride exposed to ambient air. Corrosion Science, 160, 1-19.
CrossRef - Sadeghimeresht, E., Reddy, L., Hussain, T., Huhtakangas, M., Markocsan, N., & Joshi, S. (2018). Influence of KCl and HCl on high temperature corrosion of HVAF-sprayed NiCrAlY and NiCrMo coatings. Materials & Design, 148, 17-29.
CrossRef - Eklund, J., Phother, J., Sadeghi, E., Joshi, S., & Liske, J. (2019). High-temperature corrosion of HVAF-sprayed Ni-based coatings for boiler applications. Oxidation of Metals, 91(5), 729-747.
CrossRef - Sundaresan, C., Rajasekaran, B., Varalakshmi, S., Santhy, K., Rao, D. S., & Sivakumar, G. (2021). Comparative hot corrosion performance of APS and Detonation sprayed CoCrAlY, NiCoCrAlY and NiCr coatings on T91 boiler steel. Corrosion Science, 189, 1-16.
CrossRef - Singh, A., Goyal, K., Goyal, R., & Krishan, B. (2021). Hot corrosion behaviour of different ceramics coatings on boiler tube steel at 800 C temperature. Journal of Bio-and Tribo-Corrosion, 7(1), 1-9.
CrossRef - Singh, S., Goyal, K., Bhandari, D., & Krishan, B. (2021). Hot Corrosion Behaviour of Thermal-Sprayed TiO2-Reinforced Cr2O3 Composite Coatings on T-22 Boiler Steel at Elevated Temperature. Journal of Bio-and Tribo-Corrosion, 7(3), 1-9.
CrossRef - Wang, X., He, D., Zhou, Z., Shao, W., Guo, X., & Wang, G. (2022). Hot Corrosion Behavior of Arc-Sprayed NiCrB and NiCrTi Coatings with Different Oxide Contents. Coatings, 12(4), 497.
CrossRef - Abu-warda, N., Tomás, L. M., López, A. J., & Utrilla, M. V. (2021). High temperature corrosion behavior of Ni and Co base HVOF coatings exposed to NaCl-KCl salt mixture. Surface and Coatings Technology, 418, 1-13.
CrossRef - Bhatia, R., Singh, H., & Sidhu, B. S. (2014). Hot corrosion studies of HVOF-sprayed coating on T-91 boiler tube steel at different operating temperatures. Journal of materials engineering and performance, 23(2), 493-505.
CrossRef - Singh, G., Goyal, K., & Bhatia, R. (2018). Hot corrosion studies of plasma-sprayed chromium oxide coatings on boiler tube steel at 850 C in simulated boiler environment. Iranian Journal of Science and Technology, Transactions of Mechanical Engineering, 42(2), 149-159.
CrossRef - Zhou, W., Zhou, K., Deng, C., Zeng, K., & Li, Y. (2017). Hot corrosion behaviour of HVOF-sprayed Cr3C2-NiCrMoNbAl coating. Surface and Coatings Technology, 309, 849-859.
CrossRef - N. Bala, H. Singh, S. Prakash, J. Karthikeyan (2012). Performance of cold sprayed Ni20Cr and Ni-50Cr coatings on SA-516 in actual industrial environment of a coal fired boiler, Mater. Corrosion, 63, 1–11.
CrossRef - Sadeghi, E., & Joshi, S. (2019). Chlorine-induced high-temperature corrosion and erosion-corrosion of HVAF and HVOF-sprayed amorphous Fe-based coatings. Surface and Coatings Technology, 371, 20-35.
CrossRef - Jafari, R., Sadeghimeresht, E., Farahani, T. S., Huhtakangas, M., Markocsan, N., & Joshi, S. (2018). KCl-induced high-temperature corrosion behavior of HVAF-sprayed Ni-based coatings in ambient air. Journal of Thermal Spray Technology, 27(3), 500-511.
CrossRef - Fantozzi, D., Matikainen, V., Uusitalo, M., Koivuluoto, H., & Vuoristo, P. (2019). Chlorine induced high-temperature corrosion mechanisms in HVOF and HVAF sprayed Cr3C2-based hardmetal coatings. Corrosion Science, 160, 1-11.
CrossRef - Kaushal, G., Bala, N., Kaur, N., Singh, H., & Prakash, S. (2014). Comparative high-temperature corrosion behavior of Ni-20Cr coatings on T22 boiler steel produced by HVOF, D-Gun, and cold spraying. Metallurgical and Materials Transactions A, 45(1), 395-410.
CrossRef - Kai, T. A. O., Zhou, X. L., Hua, C., & Zhang, J. S. (2009). Oxidation and hot corrosion behaviors of HVAF-sprayed conventional and nanostructured NiCrC coatings. Transactions of Nonferrous Metals Society of China, 19(5), 1151-1160.
CrossRef - Liu, S., Liu, Z., Wang, Y., & Tang, J. (2014). A comparative study on the high temperature corrosion of TP347H stainless steel, C22 alloy and laser-cladding C22 coating in molten chloride salts. Corrosion Science, 83, 396-408.
CrossRef - Jin-tao, L., Yan, L., Zhen, Y., Jin-yang, H., Ming, Z., & Gu, Y. (2018). Effect of Sulfur and Chlorine on Fireside Corrosion Behavior of Inconel 740 H Superalloy. High Temperature Materials and Processes, 37(3), 245-251.
CrossRef - El-Awadi, G. A., Abdel-Samad, S., & Elshazly, E. S. (2016). Hot corrosion behavior of Ni based Inconel 617 and Inconel 738 superalloys. Applied surface science, 378, 224-230.
CrossRef - Rani, A., Bala, N., & Gupta, C. M. (2017). Characterization and hot corrosion behavior of D-gun sprayed Cr2O3-75% Al2O3 coated ASTM-SA210-A1 boiler steel in molten salt environment. Anti-Corrosion Methods and Materials, 64(5), 515-528.
CrossRef - Thakare, J. G., Pandey, C., Mulik, R. S., & Mahapatra, M. M. (2019). Microstructure and mechanical properties of D-Gun sprayed Cr3C2-NiCr coating on P91 steel subjected to long term thermal exposure at 650° C. Materials Research Express, 6(11), 1-16.
CrossRef - Somervuori, M., Varis, T., Oksa, M., Suhonen, T., & Vuoristo, P. (2022). Comparative Study on the Corrosion Performance of APS-, HVOF-, and HVAF-Sprayed NiCr and NiCrBSi Coatings in NaCl Solutions. Journal of Thermal Spray Technology, 31, 1-17.
CrossRef - Abu-Warda, N., López, A. J., Pedraza, F., & Utrilla, M. V. (2020). Fireside corrosion on T24 steel pipes and HVOF NiCr coatings exposed to different salt mixtures. Corrosion Science, 173, 1-32.
CrossRef - Matikainen, V., Bolelli, G., Koivuluoto, H., Honkanen, M., Vippola, M., Lusvarghi, L., & Vuoristo, P. (2017). A study of Cr3C2-based HVOF-and HVAF-sprayed coatings: microstructure and carbide retention. Journal of Thermal Spray Technology, 26(6), 1239-1256.
CrossRef - Chatha, S. S., Sidhu, H. S., & Sidhu, B. S. (2012). The effects of post-treatment on the hot corrosion behavior of the HVOF-sprayed Cr3C2–NiCr coating. Surface and Coatings Technology, 206(19-20), 4212-4224.
CrossRef - Mudgal, D., Singh, S., & Prakash, S. (2015). Hot Corrosion Behavior of Bare, Cr3C2-(NiCr) and Cr3C2-(NiCr)+ 0.2 wt.% Zr Coated SuperNi 718 at 900° C. Journal of Materials Engineering and Performance, 24(1), 1-15.
CrossRef - Mudgal, D., Kumar, S., Singh, S., & Prakash, S. (2014). Corrosion behavior of bare, Cr3C2-25%(NiCr), and Cr3C2-25%(NiCr)+ 0.4% CeO2-coated superni 600 under molten salt at 900° C. Journal of materials engineering and performance, 23(11), 3805-3818.
CrossRef - Mudgal, D., Singh, S., & Prakash, S. (2015). Cyclic hot corrosion behavior of Superni 718, Superni 600, and Superco 605 in sulfate and chloride containing environment at 900° C. Metallography, Microstructure, and Analysis, 4(1), 13-25.
CrossRef - Ahuja, L., Mudgal, D., Singh, S., & Prakash, S. (2018). A comparative study to evaluate the corrosion performance of Zr incorporated Cr3C2-(NiCr) coating at 900° C. Ceramics International, 44(6), 6479-6492.
CrossRef - Goyal, K., & Goyal, R. (2020). Improving hot corrosion resistance of Cr3C2–20NiCr coatings with CNT reinforcements. Surface engineering, 36(11), 1200-1209.
CrossRef - Sidhu, V. P. S., Goyal, K., & Goyal, R. (2019). Hot corrosion behaviour of HVOF-sprayed 93 (WC-Cr3C2)-7Ni and 83WC-17Co coatings on boiler tube steel in coal fired boiler. Australian Journal of Mechanical Engineering, 17(2), 127-132.
CrossRef - Sidhu, V. P. S., Goyal, K., & Goyal, R. (2017). Comparative study of corrosion behaviour of HVOF-coated boiler steel in actual boiler environment of a thermal power plant. Journal of the Australian Ceramic Society, 53, 925-932.
CrossRef - Goyal, K., Singh, H., & Bhatia, R. (2019). Hot-corrosion behavior of Cr2O3-CNT-coated ASTM-SA213-T22 steel in a molten salt environment at 700° C. International Journal of Minerals, Metallurgy, and Materials, 26, 337-344.
CrossRef - Goyal, K., Singh, H., & Bhatia, R. (2020). Behaviour of carbon nanotubes-Cr2O3 thermal barrier coatings in actual boiler. Surface Engineering, 36(2), 124-134.
CrossRef

This work is licensed under a Creative Commons Attribution 4.0 International License.