Introduction
Magnetic materials play a crucial role in our everyday life. Permanent magnets produced from hard magnetic materials are found in home appliances such as TVs, audio systems, computers, phones, speakers, etc. The number of permanent magnets in our vehicles, for example, had grown in the recent years with the increasing demand for additional motors required for applications in starters and window lifters. Other soft magnetic materials are in transformers, power supplies, and electronic components for telecommunication applications.The wide range of applications of magnetic materials with different magnetic and physical characteristics had therefore generated a great interest in the fabrication and characterization of new magnetic materials. In this context, fabrication techniques, experimental conditions and chemical compositions of various ferrites were controlled to obtain materials with desirable properties for a wide range of applications [1 – 3].
Hexagonal ferrites (or simply, hexaferrites) form a class of magnetic materials of central importance due to their properties which can be tailored to suite specific industrial and technological applications, simple fabrication processes, chemical and thermal stability, and low cost. These ferrimagnetic oxides with hexagonal structure have a wide range of applications in permanent magnet industry, microwave devices, catalysis, and magnetic recording and data storage [1 – 10].
M-typebarium hexaferrite (also known as Ferroxdure or BaM)is a ferrimagnetic material with a melting point of 1390 °C, molecular mass 1112 g, maximum density ρ = 5.295 g/cm3and coercivity of 160–255 kA m-1. BaM hexaferrite is the most important hexaferrite in terms of production (more than 50% of the total globally manufactured magnetic materials [4]).
The unit cell of M-type barium hexaferrites is built by stacking R (BaFe6O11) and S (Fe6O8) blocks in the sequence RSR*S*, where the star denotes a block rotated by 180° about the c-axis of the hexagonal lattice [4].The unit cell therefore contains two (BaFe12O19) molecules. The S block contains two hexagonal layers of four oxygen ions in each, while the R block consists of three hexagonal oxygen layers, with one oxygen ion in the middle layer replaced substitutionally by a Ba ion. The metal ions occupy five different interstitial site: two sites in the S block (the octahedral 2a site and the tetrahedral 4f1 site), two sites in the R block (the octahedral 4f2 site and the by-pyramidal 2bsite) and one site at the R-S interfaces (the 12k octahedral site). These sublattices, their coordinations, the number of metal ions in each, and their spin directions are listed in Table 1.
Table 1: Metallic sub-lattices of M-type hexaferrite
Block
|
sublattice
|
coordination
|
ions per unit cell
|
Spindirection
|
S
|
4f1
|
Tetrahedral
|
4
|
down
|
|
2a
|
Octahedral
|
2
|
Up
|
R
|
4f2
|
Octahedral
|
4
|
Down
|
|
2b
|
Bi-pyramidal
|
2
|
Up
|
S-R
|
12k
|
Octahedral
|
12
|
Up
|
Precursor powders of barium hexaferrites were prepared by various worker using different methods of preparation such as hydrothermal synyhesis[11], sol-gel method [12,13], wet chemical method [14], ball milling [15 – 21],nitrate–citrate gel[ 22, 23], and chemical coprecipitation [24, 25].
The magnetic and electrical properties of barium hexaferrites were found to depend critically on the substitution of barium ions or iron ions by other cations and cations combinations. Trivalent metal ions, or combinations of divalent and tetravalent ions were used to substitute Fe3+ ions in the hexaferrite lattice. These included Al [15, 19,26], Ga [19, 27], (MoxZn0.4-x) [14], Mn [12], Cr [19], Ti-Ru [16], Zn–Ti [13], and Sn-Ru [18].
In the present work, we synthesized barium hexaferrites with Fe ions partially substituted by Mo6+–Zn2+ combinations. In order to maintain the cationic valence states the ratio of Mo:Znwas fixed at 1:3. The effects of the type of substitution and substitution level, and heat treatment on the structural, magnetic and physical properties of the prepared samples were investigated using x-ray diffraction (XRD), scanning electron microscopy (SEM), and vibrating sample magnetometry (VSM). The structural refinement for the prepared samples was achieved using FULLPROF software based on Rietveld refinement techniques.
Experimental
High purity (~99%) powders of BaCO3, Fe2O3, ZnO and MoO2were used as starting materials to prepare the powder precursors of Mo–Zn substituted(BaFe12-4xMoxZn3xO19)M-type hexaferrites. The barium to metal molar ratio was 1:11, and the molybdenum to zinc ratio was 1:3. Since zinc is divalent, the substitution of this combination for Fe3+ ions in these hexaferrites ensures that molybdenum has the Mo6+ valence state, which ensures the chemical neutrality of the hexaferrite.
The required amounts of the starting powders were weighed accurately and then mixed and transferred to the zirconia milling vessels. The mixture was then milled in an acetone bath (8 ml for each 5 grams of the powder) for 16 hours. The milling time was sectioned into 10 min. grinding periods interrupted by 5 min. pause periods to allow for cooling the sample and avoid overheating. The ball-to-powder mass ratio was 14:1 and the rotation speed was 250 rpm. The resulting wet powder mixture was left in the container overnight to dry at room temperature, and then the dry powder was collected in clean glass vials. An adhesive agent of aqueous solution of 2% wt. of polyvinyl alcohol (PVA) was prepared and transferred to the vial containing the milled powder and thoroughly mixed with the powder. After drying at room temperature, parts of the powder (about 1 gram each) were pressed into a discs (1.5 cm in diameter) in a stainless steel die under a 4 ton force. The discs were subsequently sintered at temperatures in the range 1100 – 1300°C.
The density for each disc was calculated by dividing the mass of the disk by its volume, and found to be independent on the level of substitution (2.7 ± 0.1 g/cm3 for all samples sintered at 1100° C). However, we noticed a tendency toward increasing the density with increasing sintering temperature, where the average density of the discs sintered at 1200° C increased to about 3.0 ± 0.1 g/cm3 and that for discs sintered at 1300° C increased up to about 3.3 ± 0.05g/cm3. This behavior could be attributed to the growth in particle size with increasing the sintering temperature, which in turn reduces the porosity of the samples.
Scanning electron microscope (SEM) system (FEI-Inspect F50/FEG)was used to investigate the micro structural characteristics of the samples. The chemical compositions of the samples were determined using the energy dispersive x-ray spectroscopy (EDX) facility available in the SEM system.
The structural characteristics of the samples were investigated using x-ray diffraction (XRD). XRD patterns of the samples were obtained in the angular range 20° – 70° using XRD 7000-Shimadzu machine with Cu-Kα radiation. The scanning step was 0.02° and the scanning rate was 1deg/min. The patterns were then analyzed using dedicated software routines (FULLPROF and Expert High Score) to determine the structural parameters of the samples.
The coercivity (Hc),remanent magnetization (Mr) and saturation magnetization (Ms) of the samples were determined from the hysteresis loops measured using a standard vibrating sample magnetometer (VSMMicro Mag 3900, Princeton Measurements Corporation). All magnetic measurements were performed at room temperature in an applied field up to 10 kOe.
Results and Analysis
Scanning electron microscopy (SEM)
Fig. 1 shows SEM images for the samples at T = 1100° C. The images indicate that the particle size and morphology does not seem to be influenced by the substitution, and show hexagonal platelets with diameters ranging from 200 nm to about 500nm for all samples under investigation. However, lighter particles with generally smaller size and different morphology was also observed, which gave the impression that we may have different phases in the samples.This was examined by EDX analysis of the spectra collected at darker (D) and lighter (L) particles of the sample with x = 0.2 (BaFe11.2Mo0.2Zn0.6O19) as an example (Fig. 2). The atomic Ba:Fe ratio in the darker particles was found to be 1:12.2 which is close to the stoichiometric ratio of BaFe12O19. On the other hand, the Ba:Mo ratio in thelighter particles was 1:1.07, which is consistent with the stoichiometric ratio of BaMoO4. These results indicate that the Mo–Zn substituted samples with BaM stoichiometry may contain a secondary barium molybdenum oxide phase coexisting with the hexaferrite major phase under these experimental conditions.
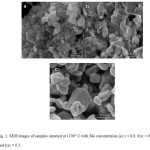 |
Fig1: SEM images of samples sintered at 1100° C with Mo concentration (a) x = 0.0, (b)x = 0.1, and (c)x = 0.2.
Click here to View Figure
|
|
Fig2: EDX patterns for darker (D) and lighter (L) particles ofBaFe11.2Mo0.2Zn0.6O19 sintered at 1100° C.
Click here to View Figure
|
This method, although does not provide an accurate quantitative analysis of the compositions of the samples due to the low concentrations of some elements in comparison with the experimental uncertainty of the technique, it is useful in checking for the possibility of having secondary phases. Therefore, a detailed structural analysis is required to identify the existing phases and their structural properties.
X-ray diffraction (XRD) measurements
Figure 3 shows the XRD patterns for all samples sintered at 1100° C. The figure indicates the development of new phases with increasing x values as evidencedby the peaks around 26.6°, 30.0°, and 35.03°. The diffraction patterns were analyzed using FULLPROF software. The pattern for the un-doped sample shows a major phase with reflections consistent with BaFe12O19 M-type hexaferrite(JCPDS: 00-043-0002) without other impurity phases. On the other hand, XRD patterns indicate that all doped samples consist of a major BaM hexaferrite phase and small amounts of other intermediate phases (ZnFe2O4 and BaMoO4). The formation of BaMoO4 phase which is consistent with the results of EDX analysis, causes deficiency in the amount of Ba (or excessive amounts of Fe and Zn) required for BaM phase, resulting in the formation of ZnFe2O4 phase.
A large number of parameters can be obtained directly from the refinement routine such as the lattice constants (a and c), cell volume V, Miller indices of the diffraction peaks (hkl), and the goodness of fit parameters. Some refined parameters resulting from fitting the experimental diffraction data are shown in Table 2.The refinement results indicates slight fluctuations of the lattice constants around a = 5.89 Å and c = 23.21 Å. The slight increase in cell volume upon substitution of iron by zinc and molybdenum could be due to partial substitution of the largerZn2+ ions (radius = 0.74 Å) for the smaller Fe3+ ions (radius = 0.49 Å)at the 4f1 tetrahedral sites[28].
Table 2. Lattice parameters (a and c), cell volume V,Bragg R-factor and RF-factor forBaFe12-4xMoxZn3xO19 samples sintered at 1100° C.
x
|
Chemical formula of sample
|
χ2
|
V (Å3)
|
a = b (Å)
|
c (Å)
|
Bragg R-factor
|
RF-factor
|
0.0
|
BaFe12O19
|
1.24
|
696.3127
|
5.8899
|
23.2066
|
0.440
|
0.600
|
0.1
|
BaFe11.6Mo0.1Zn0.3O19
|
1.15
|
698.0667
|
5.8923
|
23.2167
|
0.792
|
0.936
|
0.15
|
BaFe11.4Mo0.15Zn0.45O19
|
1.23
|
697.6312
|
5.8917
|
23.2069
|
0.545
|
0.619
|
0.2
|
BaFe11.2Mo0.2Zn0.6O19
|
1.074
|
698.4347
|
5.8933
|
23.2206
|
0.905
|
0.594
|
0.3
|
BaFe10.8Mo0.3Zn0.9O19
|
1.19
|
698.1218
|
5.8931
|
23.2119
|
0.605
|
0.566
|
In order to investigate the effect of compaction on the development of phases and crystallinity, samples in powder form were sintered at 1100° C and investigated by XRD. The refinement results indicated that these patterns are almost identical to those for disk-sintered samples, which indicates that the compaction of the powder under a 4-ton pressure did not influence the phase evolution in the samples.
The average crystallite size for each sample was calculated from the Scherrer equation [29]
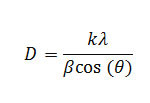
where k is a constant equals 0.9, λ = 1.542 Å, β is the peak-width at half maximum, and θ is the angular position of the peak. The average crystallite sizes for the investigated samples with x ranging from 0.0 to 0.2 are listed in Table 3.The data indicates that the crystallite size tends to decrease from about 15 nm down to about 9 nm with increasing x. This leads to the conclusion that the Mo–Zn substitution for Fe results in poorer crystallinity of the samples, probably arising from crystal defects.
Table 3.Cell volume VC and crystal size for BaFe12-4xMoxZn3xO19 s sintered at 1100° C.
x
|
Chemical formula
of ferrite
|
VC (Å3)
|
χ2
|
D
(nm)
|
0
|
BaFe12O19
|
696.3
|
1.24
|
14.6
|
0.1
|
BaFe11.6Mo0.1Zn0.3O19
|
698.0
|
1.15
|
10.7
|
0.2
|
BaFe11.2Mo0.2Zn0.6O19
|
698.4
|
1.07
|
9.1
|
To examine the effect of sintering temperature on the structure of the ferrites, all samples were resintered at 1200° C for 2 h, and their structure investigated by XRD. Fig. 4 shows X-ray patterns for resintered samples. The patterns clearly indicate that ZnFe2O4 phase disappeared in the samples, were as the BaMoO4 phase persisted throughout the whole concentration range and a newhigh-temperature phase evolved, which was identified as W-type BaZn2Fe16O27(Zn2W) phase. Baring in mind that the unit cell of the W-type phase is a combination of the unit cell of BaM and a spinel (Zn2Fe4O8) block, this phase apparently evolved according to the reaction
BaFe12O19 + Zn2Fe4O8→BaZn2Fe16O27
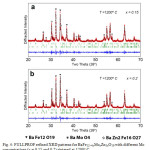 |
Fig4: FULLPROF refined XRD patterns for BaFe12−4xMoxZn3xO19 with different Mo concentrations (x = 0.15 and 0.2) sintered at 1200° C.
Click here to View Figure
|
The structural parameters for the M-type phase in these samples were derived from the FULLPROF analysis and listed in Table 4. The data indicate slight decrease in cell volume toward that of the un-substituted sample with increasing the sintering temperature. This is associated with the incorporation of Zn2+ ions in the evolving Zn2W hexaferrite rather than in the BaM hexaferrite lattice.
Table 4.Cell volume V,Bragg R-factorand RF-factorof the BaM phase in two different samples sintered at 1100 and 1200° C.
x
|
Chemical formula
of samples
|
T
|
χ2
|
V
|
Bragg R-factor
|
RF-factor
|
0.15
|
BaFe11.6Mo0.1Zn0.3O19
|
1100
|
1.23
|
697.631
|
0.545
|
0.619
|
0.15
|
BaFe11.6Mo0.1Zn0.3O19
|
1200
|
1.28
|
696.620
|
0.878
|
0.668
|
0.2
|
BaFe11.2Mo0.2Zn0.6O19
|
1100
|
1.074
|
698.435
|
0.905
|
0.594
|
0.2
|
BaFe11.2Mo0.2Zn0.6O19
|
1200
|
1.37
|
697.973
|
1.280
|
0.857
|
To further investigate the effect of sintering temperature, the sample with x = 0.15 (BaFe11.4Mo0.15Zn0.45O19)was re-sintered at a temperature 1300° C and the patterns for this sample at different temperature are shown in Fig. 5. It is evident that the BaMoO4 phase existed at all temperatures, while ZnFe2O4 disappeared completely at temperatures higher than 1200° C. At such high sintering temperatures, the reaction between the (intermediate) M-type and spinel phases is completed to form the Zn2W-type phase. Thus, we conclude that the reaction the M-typephase and the zinc spinel Zn2Fe4O8 (S) phase is favored at high temperatures (~1300° C). Our results are consistent with previously reported results [30], which indicated the presence of M-phase at low sintering temperatures, and the development of the W-phase at temperatures higher than 1200° C.
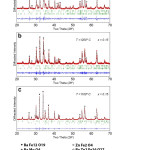 |
Fig5: FULLPROF refined XRD patterns for BaFe11.4Mo0.15Zn0.45O19 at different sintering temperature.
Click here to View Figure
|
Table 5. Cell volume VC,Bragg R-factorand RF-factor for BaFe11.4Mo0.15Zn.45O19 at different temperature
Chemical formula
of ferrite
|
Temp.
|
Number of phases:
|
|
χ2
|
VC
|
Bragg R-factor
|
RF-factor
|
(BaFe11.4Mo0.15Zn.45O19)
|
1100
|
3
|
BaFe12O19
|
1.23
|
697.63
|
0.545
|
0.619
|
|
|
BaMoO4
|
398.26
|
0.529
|
0.546
|
|
|
ZnFe2O4
|
599.55
|
0.699
|
0.632
|
1200
|
3
|
BaFe12O19
|
1.28
|
696.62
|
0.878
|
0.665
|
|
|
BaMoO4
|
396.85
|
0.830
|
0.674
|
|
|
BaZn2Fe16 O27
|
997.37
|
0.887
|
0.825
|
1300
|
3
|
BaFe12O19
|
1.45
|
703.65
|
0.781
|
0.690
|
|
|
BaMoO4
|
399.10
|
1.020
|
0.686
|
|
|
BaZn2Fe16 O27
|
997.46
|
0.645
|
0.429
|
Magnetic measurements
Fig. 6 shows the hysteresis curves for BaFe12-4xMoxZn3xO19 samples sintered at 1100° C. The curves indicate that the magnetizations do not saturate in the magnetic field range of the study. According to the law of approach to saturation, the magnetization in the high field region is dominated by magnetic domain rotation [1]. Therefore, this law was used to determine the saturation magnetization for each sample from the high field region (H > 0.8 kOe), while the coercivity and remnant magnetization were determined directly from the hysteresis loops, and the results are listed in Table 6.
As shown in Table 6 the saturation magnetization for the un-substituted sample is 70.2 emu/g,the remnant magnetization is 40.6 emu/g. The squareness ratio of about 0.58 for this sample is close to the value (0.5) for a system of randomly oriented single domain particles [3]. The saturation magnetization (Fig. 7) decreased gradually with increasing Mo concentration, recording a 19% drop in the sample with x = 0.3. However, the magnetic properties of the products are still good for permanent magnet or data storage applications.
Table 6. Saturation magnetization (Ms), remnant magnetization (Mr), coercivity (HC) and squarenes ratio (Mr/Ms) of BaFe12-4xMoxZn3xO19 samples sintered at 1100° C.
Concentration (x)
|
Chemical formula
of sample
|
Ms (emu/g)
|
HC(Oe)
|
Mr (emu/g)
|
Mr/Ms
|
0.0
|
BaFe12O19
|
70.2
|
3455
|
40.6
|
0.58
|
0.1
|
BaFe11.6Mo0.1Zn0.3O19
|
69.4
|
2594
|
37.2
|
0.53
|
0.15
|
BaFe11.4Mo0.15Zn0.45O19
|
63.6
|
2729
|
34.5
|
0.54
|
0.2
|
BaFe11.2Mo0.2Zn0.6O19
|
58.9
|
2793
|
31.3
|
0.53
|
0.3
|
BaFe10.8Mo0.3Zn0.9O19
|
57.0
|
2790
|
30.5
|
0.54
|
The initial drop in coercivity (Fig.8)of about 25% for the sample with x = 0.1 is consistent with previously reported results [14]. This behavior was associated with the substitution of the Fe3+ ions by Zn2+ ions at 4f1 sites and Mo6+ ions at 2b sites (which have the highest contribution to the magnetic anisotropy). The progressive increase of Zn2+ ions at 4f1 sites and Mo6+ ions at spin-up 2b sites is expected to result in an increase in the saturation magnetization and a further drop in coercivity, contrary to observed results. Therefore, we associate the noticeable decrease in saturation magnetization and the almost constant value of the coercivity with increasing x to the limited solubility of Zn – Mo ions in the BaM lattice, and the development of the non-magnetic (ZnFe2O4 and BaMoO4) oxide phases, which influence the saturation magnetization, but not the coercivity. In view of the reduction of the saturation magnetization for the sample with x = 0.3, the nonmagnetic oxides apparently account for 19% of the sample weight.
It is well known that the sintering temperature has an important effect on the magnetic properties, especially on the coercivity value, which is sensitive to the grain morphology [3, 31, 32]. The hysteresis loops for samples with x = 0.2 sintered at different temperatures are shown in Fig. 9. The magnetic parameters derived from these loops are listed in Table 7. The increase in saturation magnetization with increasing sintering temperature is associated with the disappearance of the nonmagnetic ZnFe2O4phase and the development of the W-phase. The sharp drop of the coercivity is in agreement with the results of Pasko et al. [30] who attributed this drop to the reaction of BaM and spinel phases to form the W-phase at temperatures higher than 1200° C. Further, the transition from a hard magnet (HC ~ 2800 Oe at 1100° C) to a soft magnet (HC ~ 300 Oe at 1300° C) could be associated with the reported spin reorientation transition from easy axis to easy plane in W-type hexaferrite. However, such transition in our sample should be confirmed by other techniques in a future work. In addition, the squarenes ratio of 0.53 for the sample sintered at 1100° C is consistent with single-domain particles randomly oriented. However, the decrease in squareness ratio at higher sintering temperatures is indicative of multi-domain particles due to particle growth at such high temperatures [30]. This multidomain structure typically results in a reduction of the coercivity [3].
Table 7. Saturation magnetization (Ms), remnant magnetization (Mr),coercivity (HC), and squareness ratio (Mr/Ms) values of ferrite samples with x = 0.2 sintered at different sintering temperatures(Ts).
Ts
|
x
|
Chemical formula
|
Ms(emu/g)
|
HC(Oe)
|
Mr(emu/g)
|
Mr/Ms
|
1100
|
0.2
|
BaFe11.2Mo0.2Zn0.6O19
|
58.9
|
2793
|
31.3
|
0.53
|
1200
|
0.2
|
BaFe11.2Mo0.2Zn0.6O19
|
65.0
|
829
|
27.2
|
0.41
|
1300
|
0.2
|
BaFe11.2Mo0.2Zn0.6O19
|
70.5
|
308
|
12.2
|
0.15
|
Conclusions
Barium hexaferrite phases were synthesized by high energy ball milling and subsequent sintering at temperatures 100, 1200, and 1300° C. Mo-Zn substitution for F3+ ions was found to result in a decrease in saturation magnetization due to the presence of secondary impurity oxide phases in samples sintered at 1100° C. Samples sintered at higher temperatures showed an increase in saturation magnetization and a drastic drop in coercivity. The proposed spin reorientation transition at room temperature in the sample with x = 0.2 sintered at 1300° C suggests that this sample has a potential application in magnetic refrigeration [30]. However, this transition should be confirmed by other techniques.
Acknowledgement
This work was supported by a generous grant from the Deanship of Scientific Research at The University of Jordan (Grant No. 1404). Thanks are due to Yousef Abu Salha and Waddah Fares (The University of Jordan) for their valuable technical assistance in XRD and SEM data collection.
References
- B.D. Cullity and C.D. Graham, Introduction to Magnetic Materials, 2nd ed. (Wiley, Hoboken, NJ, 2009).
- J. Smit and H.P.J. Wijn, Ferrites, (Philips Technical Library, Eindhoven, 1959).
- Ferromagnetic Materials, Vol. 2, E.P. Wohlfarth, ed. (North-Holland, Amsterdam, 1980).
- R.C. Pullar, Hexagonal ferrites: A review of the synthesis, properties and applications of hexaferrite ceramics, Progress in Materials Science 57 (2012)1191 – 1334.
CrossRef
- U. Ozgur, Y. Alivov, and H. Morkoc, Microwave Ferrites, Part 1: Fundamental Properties, Journal of Materials Science: Materials in Electronics 20(2009)789 – 834.
CrossRef
- B. Ul-Ain, S. Ahmed, and Y. Huang, Catalytic decomposition of N2O on cobalt substituted barium hexaferrites, Chinese Journal of Catalysis 34(2013) 1357 – 1362.
CrossRef
- T. Tanaka, T. Jitosho, and K. Yamamori, Overwriteand bit shift characteristics of Ba-ferrite floppy disks, Journal of Magnetism and Magnetic Materials134(1994) 390 – 394.
CrossRef
- D.E. Speliotis, Advanced MP++ and BaFe++ tapes, Journal of Magnetism and Magnetic Materials155(1996) 83 – 85.
CrossRef
- Z. Yang, C.S. Wang, X.H. Li, and H.X. Zeng, (Zn, Ni, Ti) substituted barium ferrite particles with improved temperature coefficient of coercivity, Materials Science and Engineering B90 (2002) 142 – 145.
CrossRef
- I.V. Zavislyak, M.A. Popov, and G. Srinivasan, A cut-off millimeter wave resonator technique for mapping magnetic parameters in hexagonal ferrites, Measurement Science and Technology. 20 (2009) 115704 (5pp).
- M. Drofenik, I. Ban, D. Makovec, A. Znidarsic, Z. Jaglicic, and D. Hanzel, The hydrothermal synthesis of super-paramagnetic barium hexaferrite particles, Materials Chemistry and Physics 127 (2011) 415 – 4
CrossRef
- C. S. Kim, S. W. Lee, and S. Y. An, Mössbauer studies of ( BaFe11.9Mn0.1O19) by a sol–gel method , Journal of Applied physics 87(2000) 6244 – 6246.
CrossRef
- Z. Haijun, L. Zhichao, M. Chengliang, Y. Xi, Z. Liangying, and W. Mingzhong, Complex permittivity, permeability, and microwave absorption of Zn- and Ti-substituted barium ferrite by citrate sol-gel process, Materials Science and Engineering B96 (2002) 289–295.
CrossRef
- G.H. Dushaq, S.H.Mahmood, I. Bsoul, H.K. Juwhari, B. Lahlouh, and M.A. AlDamen, Effects of Molybdenum concentration and valence state effects on the structural and magnetic properties of BaFe11.6MoxZn0.4-xO19 hexaferrites, Acta Metallurgica Sinica (English letters) 26 (2013) 509 – 516.
CrossRef
- J. Qiu, M. Gu, and H. Shen,Microwave absorption properties of Al- and Cr-substituted M-type barium hexaferrite, Journal of Magnetism and Magnetic Materials 295 (2005) 263–268.
CrossRef
- I. Bsoul, S. H. Mahmood, and A. Lehlooh, Structural and magnetic properties of BaFe12−2xTixRuxO19, Journal of Alloys and Compounds 489(2010)157–161.
CrossRef
- P. Sharma, R.A. Rocha, S.N de Medeiros, and A. Paesano Jr, Structural and magnetic studies on barium hexaferrites prepared by mechanical alloying and conventional route, Journal of Alloys and Compounds 443 (2007)37 – 42.
CrossRef
- A.Gonzalez-Angeles, G.Mendoza-Suarez, A. Gruskova, J. Slama, J.Lipka, and M. Papanova, Magnetic structure of Sn2+Ru4+-substituted barium hexaferrites prepared by mechanical alloying, Materials letters 59(2005) 1815_1819.
- M. Awawdeh, I. Bsoul, and S.H. Mahmood, Magnetic properties and Mössbauer spectroscopy on Ga, Al, and Cr substituted hexaferrites, Journal of Alloys and Compounds585 (2014)465–473)
CrossRef
- J. Ding, D. Maurice,W.F. Miao, P.G. McCormick, and R. Street, Hexaferrite magnetic materials prepared by mechanical alloying, Journal of Magnetism and Magnetic Materials: 150 (1995) 417–420.
CrossRef
- Ding J., Street R., and Nishio H., Magnetic properties of Ba- and Sr-hexaferrite prepared by mechanical alloying, Journal of Magnetism and Magnetic Materials 164 (1996) 385–389.
CrossRef
- S. Ounnunkad and P. Winotai,Properties of Cr-substituted M-type barium ferrites prepared by nitrate–citrate gel-autocombustion process,Journal of Magnetism and Magnetic Materials301(2006) 300–301.
CrossRef
- S. Ounnunkad, Improving magnetic properties of barium hexaferrites by La or Pr substitution, Solid State Communication 138 (2006) 472 – 475.
CrossRef
- S.R. Janasi, D. Rodrigues, F.J.G. Landgraf, and M. Emura, Magnetic properties of coprecipitated barium ferrite powders as a function of synthesis conditions,IEEE Transactions on Magnetics 36 (2000) 3327 – 3329.
CrossRef
- S.R. Janasi, M. Emura, F.J.G. Landgraf, and D. Rodrigues,The effects of synthesis variables on the magnetic properties of coprecipitated barium ferrite powders, Journal of Magnetism and Magnetic Materials 238 (2002) 168–172.
CrossRef
- D. Mishra, S. Anand, R.K. Panda and R.P. Das, X-ray diffraction studies on aluminum- substituted barium hexaferrite, Materials letters58(2004) 1147_1153.
- I. Bsoul and S.H. Mahmood, Magnetic and structural properties of BaFe12- xGaxO19 nanoparticles, Journal of Alloys and Compounds 489 (2010) 110–114.
CrossRef
- R.D. Shannon, Revised effective ionic radii and systematic studies of interatomic distances in halides and chalcogenides, Acta Crystallographica A32 (1976) 751.
- B.E. Warren, X-ray Diffraction, (Addison-Wesley, Reading, Massachusetts, 1969).
- A. Pasko, F. Mazaleyrat, M. LoBue, V. Loyau, V. Basso, M. Kupferling, C.P. Sasso, and L. Bessais, Magnetic and structural characterization of nanosized BaCoxZn2-xFe16O27 hexaferrite in the vicinity of spin reorientation transition, Journal of Physics: conference series 303 (2011) 012045 (6 pp).
CrossRef
- Y.Y. Meng, M.H. He, Q. Zeng, D.L. Jiao, S. Shukla, R.V. Ramanujan, Z.W. Liu, Synthesis of barium ferrite ultrafine powders by sol–gel combustion method using glycine gels, Journal of Alloys and Compounds 583 (2014) 220 – 225.
CrossRef
- U. Topal, H. Ozkan, K.G. Topal,Improved properties of BaFe12O19 prepared by ammonium nitrate melt technique and washed in HCl, Journal of Alloys and Compounds 422 (2006)276–278.
CrossRef
- Mahmood, S.H., et al. (2014) Magnetic Properties and Hyperfine Interactions in M-Type BaFe12-2xMoxZnxO19 Hexaferrites. Journal of Applied Mathematics and Physics, 2, 77-87. http://dx.doi.org/10.4236/jamp.2014.25011
CrossRef

This work is licensed under a Creative Commons Attribution 4.0 International License.