Ayse Kalemtas1*
, Gulsum Aydin2
and Ozge Sert1
1Bursa Technical University, Faculty of Engineering and Natural Sciences, Department of Metallurgical and Materials Engineering, Bursa, Türkiye
2Selcuk University, Faculty of Sciences, Department of Biotechnology, Konya, Türkiye
Corresponding Author E-mail: ayse.kalemtas@btu.edu.tr
DOI : http://dx.doi.org/10.13005/msri/210101
Article Publishing History
Article Received on : 19 Feb 2024
Article Accepted on : 18 Mar 2024
Article Published : 21 Mar 2024
Plagiarism Check: Yes
Reviewed by: Dr. Rakhi Bhattacharjee
Second Review by: Dr. Nitin Waghmode
Final Approval by: Dr. K. M. Garadkar
Article Metrics
ABSTRACT:
In the current study, a biowaste, chicken eggshell, was used with commercial silica powder as a raw material resource for synthesizing porous bioceramic, larnite, granules with a solid-state reaction method. This study was carried out in two primary stages. In the first step, spherical structures were obtained using a facile spherical salt bead production technique developed by Jinnapat and Kennedy. Powdered chicken eggshells and commercial silica powder were used as the inorganic starting materials to produce the spherical structures. After the shaping procedure, it was determined that spherical granules with a broad range of granule sizes, ranging from less than 2 mm to the micrometer level, were achieved. Then, in the second step, a high-temperature heat treatment was carried out to produce porous larnite granules. Performed XRD analysis revealed that the phase purity of the larnite phase was achieved at the high-temperature heat treatment done at 1250°C for 2 hours. However, it was observed that after sintering, the size of the ceramic granules slightly increased, losing their general spherical shape by sticking to each other during the sintering process. Microstructure investigations of the larnite granules revealed a fine, homogenous microstructure with a bimodal porosity distribution. The coarse porosity between the fine larnite particle clusters was determined to reach up to 15-20 mm.
KEYWORDS:
Biowaste; Calcium Silicate Ceramics; Eggshell; Larnite; Porous Bioceramics; Valorization
Copy the following to cite this article:
Kalemtas A, Aydin G, Sert O. Larnite Porous Granule Production Using Eggshell. Mat. Sci. Res. India;21(1).
|
Copy the following to cite this URL:
Kalemtas A, Aydin G, Sert O. Larnite Porous Granule Production Using Eggshell. Mat. Sci. Res. India;21(1). Available from: https://bit.ly/3INQKLq
|
Introduction
Calcium silicate ceramics are used as a biomaterial owing to their excellent characteristics such as biocompatibility, bioactivity and biodegradability 1-3. Calcium silicate ceramics are mostly used in dentistry, orthopaedics, drug delivery systems, and tissue engineering 3-6. They are also utilized in cosmetic formulations as absorbent, opacifying agent, and bulking agent 7. Calcium silicate ceramics are especially preferred in dental and orthopaedic applications due to their high bioactivity, biodegradable nature and excellent osteoconductive properties 8.
More than a few million bone graft surgeries are carried out across the globe to treat bone defects caused by disease or trauma each year 9,10. Calcium silicate ceramics are based materials release Ca+2 and Si+4 ions in the microenvironment and thus promote formation of new bone tissues 11,12. They induce cellular adhesion, promote division and differentiation of bone marrow mesenchymal stem cells 1,2. Also Si+4 ions contribute upregulation of angiogenic gene expression. Upon contact with water and environmental fluids, calcium silicate materials undergo a reaction that leads to the formation of calcium hydroxide. This reaction releases calcium and hydroxyl ions and promotes the development of bone-like apatite on their surfaces within the clinical environment. Apatite development on the surface of the material improves bonding of the material with the host bone tissue. In spite of these remarkable features, the use of calcium silicate ceramics in biomedical applications is hindered by various drawbacks including its brittleness, low strength, and rapid degradation. These limitations not only impede cell growth due to high pH levels, but also cause early bone decay before it can fully heal. One approach for enhancing the mechanical and biomedical characteristics of calcium silicate ceramics are incorporating with different ions (e.g. magnesium, silicon, zinc, and titanium). Recently using doped calcium silicate ceramics has sparked significant interest as a potential candidate for treating bone defects 8. Production of hybrid composites using ceramics or polymers is another approach to enhance mechanical characteristics of calcium silicate ceramics are.
Calcium silicate materials have emerged as a popular choice for drug carriers due to their high drug loading capabilities, pH-responsive drug release and desirable drug release characteristics 7,13,14. Calcium silicate based cements and calcium silicate based sealers are other examples of calcium silicate based materials 15,16. The utilization of calcium silicate based cements are a common practice in endodontic procedures that aim to regenerate pulp and repair damaged hard tissue, including pulp capping, apexification, and perforation repair 16. The characteristics of calcium silicate based cements such as good biocompatibility, sealing properties and ability to interact with tissues contribute to their usefulness in the aforementioned dental applications 16.
In this study, chicken eggshells and silica are used to produce larnite bioceramic synthesis. The Environmental Protection Agency has listed eggshell waste as the food sector’s 15th most significant pollution issue 17. The sustainable utilization of natural resources is of utmost importance for safeguarding the environment. It is imperative that we convert the waste produced by these resources into high-value products, thereby mitigating their impact on the environment while also promoting economic growth. We must take proactive steps to adopt resource-efficient practices that can help us achieve a cleaner and healthier planet. Recently eggshells are investigated as a very valuable waste to be used in various fields such as biodiesel production 18,19, hydroxyapatite synthesis 20,21, catalytic applications 22, and calcium silicate synthesis 23-25. In the literature, there are many studies on the synthesis of various calcium silicate ceramics using natural wastes such as eggshells 23-25, sea shells 26, and rice husks 24. Choudhary et al. 24 used rice husk as a silica source and eggshell as a calcium oxide source to produce calcium silicate bioceramics. Choudhary et al. 24 synthesized wollastonite at 1100°C and the optimal calcination temperature for forsterite was found to be 1300°C. Kaou et al. 23 used spark plasma sintering (SPS) to produce calcium silicate ceramic using chicken eggshell and silica gel as the starting raw materials.
The two essential objectives of the current study are (i) to synthesize calcium silicate by using eggshell and silica starting materials and (ii) to apply a facile spherical salt bead production technique developed by Jinnapat and Kennedy 27 for the production of porous larnite granules.
Material and Method
Calcium silicate porous bioceramic granules were produced using chicken eggshells and silica (Esan, Türkiye). Waste chicken eggshells were supplied from a local restaurant in Bursa. Eggshells were washed and mechanically cleaned, and the inner tissues of the eggshells were removed and then dried in an oven. Then, the eggshells were ground with a disc mill at 1000 rpm for 3 minutes to achieve fine eggshell powder. Silica powder was milled in a planetary ball mill in an aqueous environment at 300 rpm for four hours to decrease the particle size of the starting silica powder. After planetary ball milling, the sample was dried in an oven.
Eggshell powder and silica-containing mixture (EPS) was milled in a planetary ball mill in an aqueous environment at 300 rpm for one hour to achieve homogenous mixture of the desired calcium silicate ceramic composition at a stoichiometric ratio. After attaining a homogenous slurry via an aqueous milling procedure, the slurry was dried in an oven. The dried sample was ground in an agate mortar to achieve a relatively fine and homogeneous powder mixture. Bioceramic granule production was performed using the novel process (Fig. 1) that Jinnapat and Kennedy 27 developed to produce spherical salt beads, with minor revisions. In the current study, EPS was used instead of salt, and the applied sintering procedure was changed accordingly to achieve the desired calcium silicate phase content.
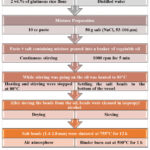 |
Figure 1: Flow chart for the novel process that Jinnapat and Kennedy 27developed to produce spherical salt beads
Click here to View Figure
|
Green ceramic granules were sintered at 1250°C for 2 hours with a heating and cooling rate of 5°C/min at atmospheric conditions. Microstructural investigation of the samples were carried out with a scanning electron microscope (SEM, Zeiss/Gemini 300). Phase content of the raw chicken eggshell and sintered ceramic granules were performed with X-ray diffraction (XRD, Bruker D8) using Cu−Kα radiation (l=1.5406Å). Infrared transmission spectra of the eggshells powder was recorded by an FT-IR spectrometer (Thermo Scientific Nicolet IS50, USA) in the wave number range of 400 to 4000 cm−1 with 2 cm−1 resolution.
Results and Discussion
Scanning electron microscopy images of the milled silica powder are given in Fig. 2. It was determined that the particle size of the obtained silica powder was smaller than 10 mm after planetary ball milling of the coarse silica powders. It was observed that generally, a homogenous and relatively wide particle size distribution, approximately 10 mm to < 150 nm, was achieved after wet milling.
Scanning electron microscopy images of the grounded eggshells powder are given in Fig. 3. Dry grounded eggshells powder particle size distribution was approximately 150 mm to < 100-150 nm (Fig. 3). It was determined that the average particle size and distribution of the powdered eggshells are significantly coarser and wider when compared with the milled silica powder.
The X-ray diffraction pattern of the powdered chicken eggshell given in Fig. 4 revealed the existence of only a calcite phase in the structure. In previous studies, similar results were reported for the phase analysis of the untreated eggshells 24,28,29. Choudhary et al. 24 produced calcium silicate bioceramics using rice husk as a silica source and eggshell as a calcium oxide source, and they reported that the CaCO3 phase was the only phase that existed in the grounded eggshell sample. The same phase content for the eggshell was reported by Engin et al. 28. They investigated the effect of temperature on the structure of the eggshells and determined that the eggshells almost completely decomposed when the heat treatment was carried out at 900° for 1 hour 28.
Kaou et al. 23 used SPS to produce calcium silicate ceramic using chicken eggshell and silica gel as the starting raw materials. They reported that 1408, 873, and 712 cm-1 were the major absorption peaks 23 in the FTIR analysis of the raw chicken eggshell sample, which are very close to the determined wavenumbers in this study (Fig. 5). In the current research, FTIR analysis of the dried eggshell powder revealed that 1409, 872, and 712 cm-1 are the major absorption peaks (Fig. 5). These three absorption peaks indicated asymmetric stretch, out-of-plane bend, and in-plane bend vibration modes, respectively, for CO2−3 molecules which exist in the structure of calcium carbonate. Hossain et al. 30 carried out crystallographic investigation of the waste eggshells and they reported similar FTIR results. They reported that the wavenumber of 710 ( ν4 in-plane bend) and 875 cm−1 (ν2 out-of-plane bend) peaks have appeared for the existence of calcium carbonate phase 30. It is reported that these two peaks are significant for quantitatively estimating calcium carbonate amount in a mixture 30,31. Characterization of the eggshell powders using XRD (Fig. 4) and FTIR (Fig. 5) analysis confirmed the calcium carbonate phase as the primary content in the eggshell powders as reported in the literature 28,32.
Fig. 6 shows macroscopic images of the green granules and sintered ceramic granules. The green granules, which were produced using Jinnapat and Kennedy’s process 27, are spherical in shape and exhibit a broad range of bead sizes, ranging from less than 2 mm to the micrometer level (Fig. 6-a). After sintering at 1250°C for 2 hours, the organic content of the beads was completely removed, and the size of the ceramic beads slightly increased, losing their general spherical shape by sticking to each other during the sintering process (Fig. 6-b). Thus, the sintered beads were called as granules.
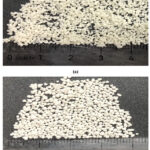 |
Figure 6: Macroscopic images of the (a) green ceramic granules produced according to the process developed by Jinnapat and Kennedy 27 and (b) sintered ceramic granules.
Click here to View Figure
|
Engin et al. 28 studied the effect of the applied heat treatment temperature on the phase content of the eggshells. Their XRD analysis revealed that when the applied heat temperature reached 700°C and heat treated the eggshells for 1 hour at this temperature, CaO formation was determined according to the reaction (1) given below. They reported that the amount of CaO formation increases when the temperature increases, whereas the calcium carbonate phase content decreases after 700°C. They reported that the eggshells almost completely decomposed at 900° for 1 hour, and CaO formation was completed.
CaCO3 ® CaO + CO2 (1)
Eggshells derived CaO can be used in various bioceramic synthesis such as calcium silicates 23-25 and hydroxyapatite 20,21. Choudhary et al. 24 used rice husk as a silica source and eggshells as a calcium oxide source to produce calcium silicate bioceramics such as diopside, forsterite, and wollastonite by utilizing a solid-state method. They extracted silica, a highly valuable resource, from rice husks through a series of chemical processes, starting with decomposing the rice husks in a furnace. After this step, an alkali treatment was applied. Then, an acid precipitation step was carried out. Once extraction was done, obtained silica was reacted with raw eggshells at elevated temperatures to synthesize desired bioceramic products. The TG-DSC analysis revealed that diopside, wollastonite, and forsterite crystallization temperatures were determined as 870°C, 883°C, and 980°C, respectively 24. It was reported that while the phase purity of the wollastonite phase was achieved at 1100°C. However, diopside and forsterite phases still contained some secondary phases even after applied calcination procedure at 1250°C and 1300°C, respectively 24. In this study, larnite (Ca2SiO4) phase was achieved after heat treatment at 1250°C for 2 hours (Fig. 7). Performed XRD analysis revealed that larnite phase was the only phase exist in the system after the carried out high temperature heat treatment (Fig. 7). With twice the calcium concentration of wollastonite, larnite has been reported to possess better bioactivity than wollastonite 33,34.
Scanning electron microscopy investigations of the larnite granules revealed a fine, homogenous microstructure with a bimodal porosity distribution (Fig. 8). It was determined that the coarse porosity between the fine particle clusters can reach up to 15-20 mm. During the high-temperature heat treatment process, fine and coarse porosity formation took place with the removal of the organics used in the shaping step to achieve the granule morphology (Fig. 8). This microstructure was found to be fine and homogenous, with the particle clusters being evenly distributed. The presence of such a distinctive structure opens up opportunities for a wide range of scientific and industrial applications that demand high open porosity-containing materials.
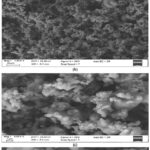 |
Figure 8: Fracture surface SEM images of sintered calcium silicate granules at various magnifications. (a) 500X, (b) 1kX, (c) 5kX and (d) 10kX.
Click here to View Figure
|
Conclusion
The world’s natural resources are finite and are being depleted rapidly. Simultaneously, the amount of waste generated annually is increasing significantly. It is important to convert natural resource waste into high-value products for efficient utilization of the resources and environmental protection.
The Environmental Protection Agency has listed eggshell waste as the food sector’s 15th most significant pollution issue 17. Synthesis of bioceramics using eggshell waste can be an attractive solution for producing economical biomaterials in a green environment.
In this study, a biowaste, chicken eggshell, was used as a calcium oxide source instead of a synthetic commercial starting material with commercial silica powder to synthesize larnite bioceramic.
A stoichiometric calcium oxide/silica ratio was used to achieve desired ceramic product, larnite.
A facile spherical salt bead production technique developed by Jinnapat and Kennedy 27 is successfully applied for the production of millimeter-sized porous larnite bioceramic granules.
XRD results revealed that larnite bioceramic synthesis by using eggshell and silica starting materials was achieved after applied heat treatment at 1250°C for 2 hours.
Microstructure investigations of the larnite granules revealed a fine, homogenous microstructure with a bimodal porosity distribution. The coarse porosity between the fine larnite particle clusters was determined to reach up to 15-20 mm.
Porous larnite bioceramic granules can be used in various ceramic, polymer, and metal matrix composite systems to develop bioproducts such as composite scaffolds.
Acknowledgments
The authors gratefully acknowledge to Bursa Technical University, Scientific Research Project Unit. Özge SERT is supported by Turkish Council of Higher Education (YÖK) 100/2000 PhD Scholarship.
Conflict of Interest
None to decalare
Funding source
Bursa Technical University, Scientific Research Project Unit (Project No: 210T008).
References
- Ding S-J, Shie M-Y, Hoshiba T, Kawazoe N, Chen G, Chang H-C. Osteogenic differentiation and immune response of human bone-marrow-derived mesenchymal stem cells on injectable calcium-silicate-based bone grafts. Tissue Engineering Part A. 2010;16(7):2343-2354.
CrossRef
- Piedad N, García-Bernal D, Cragnolini F, Velasquez P, Meseguer-Olmo L. The effects of Ca2SiO4–Ca3(PO4)2 ceramics on adult human mesenchymal stem cell viability, adhesion, proliferation, differentiation and function. Materials Science and Engineering: C. 2013;33(7):4009-4020.
CrossRef
- Srinath P, Abdul Azeem P, Venugopal Reddy K. Review on calcium silicate‐based bioceramics in bone tissue engineering. International Journal of Applied Ceramic Technology. 2020;17(5):2450-2464.
CrossRef
- Venkatraman SK, Swamiappan S. Review on calcium‐and magnesium‐based silicates for bone tissue engineering applications. Journal of Biomedical Materials Research Part A. 2020;108(7):1546-1562.
CrossRef
- Liu Z, He X, Chen S, Yu H. Advances in the use of calcium silicate-based materials in bone tissue engineering. Ceramics International. 2023;49(11):19355-19363.
CrossRef
- Ribas RG, Schatkoski VM, do Amaral Montanheiro TL, et al. Current advances in bone tissue engineering concerning ceramic and bioglass scaffolds: A review. Ceramics International. 2019;45(17):21051-21061.
CrossRef
- Zhu Y-J, Guo X-X, Sham T-K. Calcium silicate-based drug delivery systems. Expert opinion on drug delivery. 2017;14(2):215-228.
CrossRef
- No YJ, Li JJ, Zreiqat H. Doped calcium silicate ceramics: a new class of candidates for synthetic bone substitutes. Materials. 2017;10(2):153.
CrossRef
- Giannoudis PV, Dinopoulos H, Tsiridis E. Bone substitutes: an update. Injury. 2005;36(3):S20-S27.
CrossRef
- Xue N, Ding X, Huang R, et al. Bone tissue engineering in the treatment of bone defects. Pharmaceuticals. 2022;15(7):879.
CrossRef
- Zhou P, Xia D, Ni Z, et al. Calcium silicate bioactive ceramics induce osteogenesis through oncostatin M. Bioactive Materials. 2021;6(3):810-822.
CrossRef
- Li H, Chang J. Stimulation of proangiogenesis by calcium silicate bioactive ceramic. Acta biomaterialia. 2013;9(2):5379-5389.
CrossRef
- Huang C-Y, Huang T-H, Kao C-T, Wu Y-H, Chen W-C, Shie M-Y. Mesoporous calcium silicate nanoparticles with drug delivery and odontogenesis properties. Journal of endodontics. 2017;43(1):69-76.
CrossRef
- Islam MS, Choi HN, San Choi W, Lee H-J. Polyelectrolyte-mediated hierarchical mesoporous calcium silicates: a platform for drug delivery carrier with ultrahigh loading capacity and controlled release behavior. Journal of Materials Chemistry B. 2015;3(6):1001-1009.
CrossRef
- Guivarc’h M, Jeanneau C, Giraud T, et al. An international survey on the use of calcium silicate-based sealers in non-surgical endodontic treatment. Clinical Oral Investigations. 2020;24:417-424.
CrossRef
- Eskandari F, Razavian A, Hamidi R, Yousefi K, Borzou S. An updated review on properties and indications of calcium silicate-based cements in endodontic therapy. International Journal of Dentistry. 2022;2022
CrossRef
- Waheed M, Yousaf M, Shehzad A, et al. Channelling eggshell waste to valuable and utilizable products: a comprehensive review. Trends in Food Science & Technology. 2020;106:78-90.
CrossRef
- Odetoye T, Agu J, Ajala E. Biodiesel production from poultry wastes: Waste chicken fat and eggshell. Journal of Environmental Chemical Engineering. 2021;9(4):105654.
CrossRef
- Attari A, Abbaszadeh-Mayvan A, Taghizadeh-Alisaraei A. Process optimization of ultrasonic-assisted biodiesel production from waste cooking oil using waste chicken eggshell-derived CaO as a green heterogeneous catalyst. Biomass and Bioenergy. 2022;158:106357.
CrossRef
- Khalid M, Jikan SSB, Adzila S, et al. Synthesis and characterizations of hydroxyapatite using precursor extracted from chicken egg shell waste. Biointerface Res Appl Chem. 2022;12(4):5663-5671.
CrossRef
- Shi D, Tong H, Lv M, et al. Optimization of hydrothermal synthesis of hydroxyapatite from chicken eggshell waste for effective adsorption of aqueous Pb (II). Environmental Science and Pollution Research. 2021;28(41):58189-58205.
CrossRef
- Awogbemi O, Inambao F, Onuh EI. Modification and characterization of chicken eggshell for possible catalytic applications. Heliyon. 2020;6(10)
CrossRef
- Kaou MH, Furkó M, Rachid BZH, Balázsi K, Balázsi C. Morphological and structural evaluation of spark plasma sintered calcium silicate ceramics. International Journal of Applied Ceramic Technology. 2024;
CrossRef
- Choudhary R, Venkatraman SK, Bulygina I, et al. Biomineralization, dissolution and cellular studies of silicate bioceramics prepared from eggshell and rice husk. Materials Science and Engineering: C. 2021;118:111456.
CrossRef
- Anjaneyulu U, Sasikumar S. Bioactive nanocrystalline wollastonite synthesized by sol–gel combustion method by using eggshell waste as calcium source. Bulletin of Materials Science. 2014;37:207-212.
CrossRef
- Pahlevani F, Sahajwalla V. Synthesis of calcium silicate from selective thermal transformation of waste glass and waste shell. Journal of Cleaner Production. 2018;172:3019-3027.
CrossRef
- Jinnapat A, Kennedy A. The manufacture of spherical salt beads and their use as dissolvable templates for the production of cellular solids via a powder metallurgy route. Journal of Alloys and Compounds. 2010;499(1):43-47.
CrossRef
- Engin B, Demirtaş H, Eken M. Temperature effects on egg shells investigated by XRD, IR and ESR techniques. Radiation Physics and Chemistry. 2006;75(2):268-277.
CrossRef
- Naemchanthara K, Meejoo S, Onreabroy W, Limsuwan P. Temperature effect on chicken egg shell investigated by XRD, TGA and FTIR. Advanced Materials Research. 2008;55:333-336.
CrossRef
- Hossain MS, Jahan SA, Ahmed S. Crystallographic characterization of bio-waste material originated CaCO3, green-synthesized CaO and Ca (OH) 2. Results in Chemistry. 2023;5:100822.
CrossRef
- Reig FB, Adelantado JG, Moreno MM. FTIR quantitative analysis of calcium carbonate (calcite) and silica (quartz) mixtures using the constant ratio method. Application to geological samples. Talanta. 2002;58(4):811-821.
CrossRef
- Choudhary R, Koppala S, Swamiappan S. Bioactivity studies of calcium magnesium silicate prepared from eggshell waste by sol–gel combustion synthesis. Journal of Asian Ceramic Societies. 2015;3(2):173-177.
CrossRef
- Gou Z, Chang J, Zhai W. Preparation and characterization of novel bioactive dicalcium silicate ceramics. Journal of the European Ceramic Society. 2005/06/01/ 2005;25(9):1507-1514.
CrossRef
CrossRefdoi:https://doi.org/10.1016/j.jeurceramsoc.2004.05.029
- Gou Z, Chang J. Synthesis and in vitro bioactivity of dicalcium silicate powders. Journal of the European Ceramic Society. 2004;24(1):93-99.
CrossRef

This work is licensed under a Creative Commons Attribution 4.0 International License.