Introduction
The pharmaceutical industry is a prime illustration of how scientists from different fields can successfully collaborate to create novel and potent product. After being discovered, it may take decades to finish all required clinical trials and receive regulatory approval for the new a drug or excipient. Orally administered therapeutic agents are currently the simplest, most practical, and most user-friendly method of medication delivery. When it comes to patient convenience, an oral drug formulation poses a challenge to the researchers, who must create drug delivery systems that allow a particular medicine to achieve a certain pharmacokinetic profile1. There has been a lot of interest in recent years in the creation and application of tablets that, once taken, release the medication gradually but steadily in the gastrointestinal tract to produce a long-lasting therapeutic impact2,3. Extended release, sustained release, Slow release, timed release, prolonged release, respiratory dose forms and depots are some of the names given to these tablets. Extended release formulations are more appealing than traditional dosage forms for a number of reasons, including their continuous bioavailability, ability to decrease the periodicity of drug administration by extending the duration of effective blood levels, improved drug distribution, decreased peak-through concentration fluctuation, and decreased side effects4,5. One of the simplest methods for producing extended-release dosage forms is the matrix tablet, in which the medication is distributed throughout a polymer matrix6,7. Nowadays, the most common synthetic, biodegradable polymers in human medicine are aliphatic polyesters made from polylactic acid (PLA) and poly glycollic acid (PGA)8,9. A polymer made from biocompatible monomers is preferable for use in biomedical applications. Pharmaceutical industries use maleic acid to stabilize some drugs by preparing acid addition salts. Citric acid is found in a number of plant species (citrus fruits) and is broadly used in pharmaceutical preparations and food products, mainly to regulate the pH of solutions10. On the other hand, propane-1,2-diol is being commonly used as an extractant, preservative and solvent in a range of pharmaceutical products and is mostly considered as a comparatively non-hazardous material10. Though, the biocompatibility of a monomer does not confirm the same for its polymer but we can have an idea from it.
Selecting these pharmaceutically accepted monomers, Poly[(maleic acid)-co-(propane-1,2-diol)-ran-(citric acid)] (PMPC) has been prepared and studied as a polymer matrix for extended release of drugs. Its synthesis, characterization, bio-degradation, and drug release characteristics will be reported in this article. On the other hand, dichlofenac sodium is a non-steroidal, anti-inflammatory and anti-pyretic drug which is available as enteric coated oral formulation in the market. However, enteric coatings do not provide the benefits of extended release. We targeted to devise a drug carrier that will provide both enteric properties and extended release characteristics for such kind of drugs. So selecting dichlofenac sodium a model drug, its in-vitro release from polymer-matrix tablets will also be discussed.
Materials and Methods
Citric acid, propane-1,2-diol, and maleic acid, were analytical reagent grade chemicals from E. Merck India. Analytical reagent grade anhydrous FeCl3, used as catalyst, was freshly sublimed prior to use. Diclofenac sodium samples in pure form were acquired from Kemiko Pharmaceuticals Ltd. Rajshahi, Bangladesh.
Synthesis of the polymer
The polycondensation reaction (between the respective monomers in the desired ratio) was conducted in a Dean-Stark apparatus in presence of xylene. Anhydrous FeCl3 (about o.4% of the total weight) was employed as the catalyst. The reaction was carried out for around six hours in 135-1400C. After being dissolved in acetone, PMPC was extracted from the reaction chamber and purified by precipitation with water acting as a non-solvent. PMPC was then stored in a desiccator after being vacuum dried at 600C.
Maleic acid, propane-1,2-diol (acid/diol mole ratio = 1), and 0.1, 0.2, 0.3, 0.4, and 0.5% of citric acid were used to create five polymer samples (I-V) based on the total weight of the monomers, respectively. It was anticipated that random cross-linking would occur all over the structure due to citric acid.
Characterization
FTIR spectra, equilibrium swelling in rectified spirit and water, molecular weights, soil burial biodegradation, and solubility in common organic solvents at ambient temperature were used to characterize the co-polyesters. Care was given to prevent any solvent loss during the gravimetric measurements for equilibrium swelling. The PMPC sample was powered cryogenically and its FTIR spectrum on KBr pellet was noted by a Shimadzu FTIR-8400 spectrophotometer (400-4000 cm-1). Thermogravimetric (TGA) data were documented by a Perkin Elmer simultaneous thermal analyzer,
Molecular Weight Determination
The fractional precipitation technique was performed at (20±1)°C. For this purpose, acetone was used as a solvent while water as a non-solvent. Viscosity measurements (Mn) and end group analysis (Mv) were used to determine the molecular weights of these fractions. A precisely weighed amount of the polymer sample (less than 0.8 g) was dissolved in ethylacetate for end group analysis. Using phenolphthalein as an indicator, this was titrated against a standard 0.1(N) alcoholic potassium hydroxide solution. The end point was the appearance of a slightly pink color. However, using acetone as a solvent, the intrinsic viscosities of these fractions were measured by Ostwald’s viscometer.
Hydrolytic Degradation Study
To assess the degradation properties of the synthesised co-polyester in solutions with different pH values, hydrolytic degradation was performed. Both acidic and alkaline solutions with varying pH values were used for this investigation. These solutions were made with sodium carbonate and hydrochloric acid. The degradation of the polyester was thought to be indicated by the change in pH between the solution containing the PMPC sample and the blank solution over time.
Preparation of Drug-Polymer Matrix Tablets11
With diclofenac sodium serving as the model drug, PMPC was employed as a drug carrier in matrix form. Both the drug and the polymer were precisely weighed. Next, pure diclofenac sodium was mixed in the melted polymer so that 50 mg of the drug was present in each tablet. Following cooling and meshing, the granules were compressed into tablet shapes using a single punch tablet machine. For lubricating the machine’s punch and die surfaces, magnesium stearate was utilized.
In-vitro Drug Release Study of the Matrix Tablets 12-13
The purpose of this investigation was to determine the release kinetics of diclofenac sodium dispersed in the co-polyester matrix. The dissolution medium was 1000 ml of phosphate buffer (pH 7.4) stirring at 37°C. At 30-minute intervals, a 5 ml aliquot portion of the medium was taken out, and its absorbance was measured (after appropriate dilution if needed) at 274 nm ( λmax of diclofenac sodium) using a Shimadzu UV-VIS (Model: U-1800) spectrophotometer. The volume loss of the medium was promptly made up with the same amount of fresh medium that had been preheated at 37°C±0.5°C. The concentrations of the released medication were then determined by comparing them to standard curves made for the pure drug in a pH 7.4 phosphate buffer solution in the relevant concentration range. This test was performed on five drug-polymer matrix tablet samples, with variances in concentration levels falling within 1%.
Soil Burial Biodegradability Test
A soil burial test was evaluated to mimic the natural biodegradation of the said polyester. This test was conducted, with a few minor adjustments, in accordance with Kumar et al14. In order to carry out this test, several 1000 ml beakers with almost similar diameters were filled with gray color garden soil. The soil in the beakers was thoroughly mixed and the polymer sample weighing about 5g and uniform rectangular shape was placed in the middle of each of the beakers at a depth of 2 inches. The soil’s pH was between 7 and 7.5. Regular sprinkling of water keeps the soil’s relative humidity high. About 25°C was the typical temperature in the laboratory. Every sample has its own unique number on the beakers. The sample was then taken out at regular intervals of 15 days, gently cleaned with water to get rid of any soil that had stuck to its surface, and vacuum-dried at 40° C until a constant weight was achieved. The weight loss over time of the sample was measured as a sign of its biodegradation. Using the following formula, weight losses were computed.
% weight loss = (Wi-Wf)/Wi×100%,
Where Wi is the weight of sample prior soil burial and Wf is the weight of sample following soil burial. Three sets of data were so collected and averaged.
Results and Discussion
Synthesis
Both prpane-1,2-diol and maleic acid are bi-functional monomers. They were polycondensed in a 1:1 stoichiometric ratio. The third reactant, citric acid, has one tertiary hydroxyl group and three carboxylic groups. Citric acid was utilised as a cross-linking agent in trace amounts, ranging from 0.1 to 0.5% of the reactants’ total weight. The following is the predicted co-polyester structure:
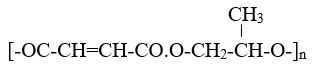
On the other hand, the structure would randomly cross-link due to the presence of citric acid. At room temperature, the solid, slightly sticky, brown PMPC samples were synthesized.
Characterization
Solubility
Good solvents for PMPC include acetone, a toluene and ethanol mixture (toluene: ethanol=3:1), and ethylacetate. Nevertheless, it dissolves negatively in toluene and ethanol. However, prevalent non-solvents for this polyester include water, benzene, xylene, formic acid, rectified spirit, etc.
Swelling Behavior
The equilibrium swelling values of the five PMPC samples (I-V) in rectified spirit and water at room temperature are shown in Table 1. It is evident that sample-V, which contains 0.5 wt% citric acid in each of the two solvents, has the lowest equilibrium swelling value. For a set of chemically equivalent cross-linked polymers, the degree of swelling at a specific temperature and solvent is inversely proportional to the network’s crosslink density15. Thus, the swelling study’s findings show that the molecular weight and crosslink density follow the order V > IV > III > II > I.
Higher than 0.5% citric acid will undoubtedly enhance the co-polyester domain’s crosslink density, decreasing its solubility in typical organic solvents and making it more challenging to work with in large-scale pharmaceutical applications.
Once more, the rectified spirit > water is the order of the equilibrium swelling values in the two solvents. The reason for this is most likely that rectified spirit has superior solvent properties compared to water. For the ensuing tests, sample-V was chosen based on its swelling behavior.
Table 1: Equilibrium swelling of PMPC in water and in rectified sprit at room temperature.
Polymer (MCPC) |
Acid/diol
mole ratio |
% of
Citric acid |
% of equilibrium swelling (w/w) in |
Water |
Rectified sprit |
I |
1 |
0.1 |
95.0 |
111.0 |
II |
0.2 |
90.0 |
108.0 |
III |
0.3 |
87.0 |
103.0 |
IV |
0.4 |
84.0 |
97.0 |
V |
0.5 |
80.0 |
89.0 |
Molecular Weight
End group analysis
Using end group analysis, the number average molecular weight (Mn) of each fraction of PMPC was measured. The values for the different fractions were 22945, 19530, 17253, 13638, and 11220, respectively. Table 2 displays the weight percentages (wt%) of fractionated polymer in unfractionated polymer samples. These results depict that the fraction no.-iii of the sample (mol. wt. 17253) has the maximum quantity in the unfractionated polymer sample.
Table 2: Molecular weight distribution of fractionated PMPC in un-fractionated polymer.
Polymer
fraction no. |
Weight % of
fraction |
Mol. wt. by end group
analysis ( ) |
i |
8.9 |
22945 |
ii |
25.5 |
19530 |
iii |
36.4 |
17253 |
iv |
18.6 |
13638 |
v |
10.6 |
11220 |
Viscosity method
The logarithms of the intrinsic viscosities of these polymer fractions were plotted against the logarithms of their number average molecular weights (Mn) yielding a linear curve whose intercept and slope gave the values of the Mark-Hauwink16 constants ‘K’ and ‘a’, respectively.
Table 3: Molecular weights of PMPC obtained by end group analysis and the same obtained by viscosity method
Fraction no. |
Intrinsic
viscosity,[η]
ml/g
(from graph) |
K x 103
(from graph)ml/g |
a
(from graph) |
Molecular weight by |
End group
analysis(Mn) |
Viscosity method(Mv) |
i
ii
iii
iv
v |
24.87
22.28
20.37
17.31
15.29 |
15.80 |
0.73 |
22945
19530
17253
13638
11220 |
23960
20609
18228
14585
12304 |
According to the Mark-Hauwink equation, the correlation between viscosity average molecular weight (Mv) and intrinsic viscosity is as follows:

Table-3 also shows the comparison between the values of the molecular weights of different fractions of this polymer sample measured using end group analysis and the same derived by the viscosity method, and it was found that the molecular weight obtained by viscometry (Mv) is greater than that obtained by end group analysis (Mn).
FTIR Characterization
The broad band indicating the -OH group at 3100-3500cm-1 in the diol spectrum nearly disappeared in the PMPC spectrum (Figure 1). The >C=O stretching frequency in the maleic acid spectrum shifted from 1700-1710 cm-1 to 1714.7 cm-1, and a new band signifying ester linkage developed at 1155 cm-1 in the polyester spectrum. All of them suggest the interaction between -OH and -COOH groups, producing ester linkages in the sample.17.
TGA Analysis
TGA analysis was done to figure out the thermal stability of the copolyester under consideration. No significant weight loss was observed up to 1500C from thermogravimetric analysis (Figure-2).
Elemental Analysis
Calculated for the predicted structure: C = 53.85%, H = 5.12%, and obtained for co-polyester sample-V: C = 53.21% and H = 5.45%. The percentage composition of the sample derived from calculation is consistent with that found by analysis. The product is accordingly validated.
Soil Degradation Test
This test was conducted to determine the biodegradability of the aforementioned polymer in ideal and realistic environmental conditions. Figure 3 depicts the steady weight loss of the polymer buried in soil, and after 55 days, it eventually mixed with the surrounding soil. This indicates the total decay of the polyester sample in the soil 18, 19. This weight loss is presumably owing to the dirt present in the soil, moisture and humidity, and the hydrophilic character of the co-polyester, which causes swelling, leading to the formation of micro cracks that create a favourable scenario for soil-borne microbes to attack the polymer network.20, 21. As these newly generated micro cracks open, the deterioration process progresses.
Hydrolytic Degradation Study
The hydrolytic degradation investigation (Figures 4 and 5) at room temperature revealed that the co-polyester sample progressively deteriorated in the basic medium (pH>7.0) but stayed nearly intact in the acid medium (pH 1.2–6.0). The polymer sample barely swells in an acidic area. However, in an alkaline environment, it swells and the ester bond is hydrolyzed, which causes the pH of the solutions containing the polymer to significantly drop over time. To determine the potential use of this polyester in controlled release oral formulations in the intestine, where the human body has a basic pH area, its pH responsive and time-dependent breakdown nature should be evaluated. The co-polyester was found to be unable to create a soft, continuous film over a glass substrate, though. Hence, instead of being used as an enteric coating material, it was more effective as a drug carrier for extended release drug-polymer matrix tablets. It is necessary to look into its in-vitro drug release behavior in this regard.
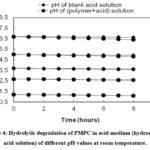 |
Figure 4: Hydrolytic degradation of PMPC in acid medium (hydrochloric acid solution) of different pH-values at room temperature.
Click here to View Figure |
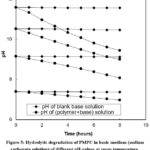 |
Figure 5: Hydrolytic degradation of PMPC in basic medium (sodium carbonate solution) of different pH-values at room temperature.
Click here to View Figure |
In-vitro Drug Release Study of the Matrix Tablets (Drug Dissolution Study)
An in vitro release kinetics analysis was conducted by immersing a diclofenac sodium-loaded PMPC polymer matrix tablet in a phosphate buffer solution with a pH of 7.4 at 37°C (simulated intestinal fluid)12,13. It was seen that within the first ten hours, the tablet steadily lost size while maintaining its physical integrity and shape. The matrix then started to break up into tiny bits, which, over the course of the following four hours, totally dissolved in the buffer. Figure 6 displays the cumulative release and percent release/hour of diclofenac sodium from drug-loaded polymer matrix tablets in simulated intestinal fluid. It shows a steady rate of release (zero order) for the first 10 hours and approximately 90% of the drug released in the first 12 hours; after that, the drug release was minimal.
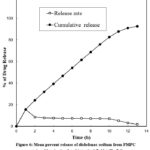 |
Figure 6: Mean percent release of diclofenac sodium from PMPC matrix tablets in simulated intestinal fluid (pH =7.4).
Click here to View Figure |
The tablet’s reduced thickness, preservation of its structural integrity, and zero order drug release kinetics indicate that drug release occurs primarily through tablet surface erosion and that drug diffusion is negligible. This statement is supported by other researchers22. The unbound drug particles on the tablet surface enter the solution as soon as it is placed in the buffer, which results in a high rate of release within the first hour. Other researchers have also noted such high initial rate of medication release23-26.
Conclusion
In this work, soil burial biodegradation and pH responsive degradation behavior of Poly[(maleic acid)-co-(propane-1,2-diol)-ran-(citric acid)] were reported. The hydrolytic degradation investigation revealed that the co-polyester sample stayed nearly intact in acidic medium (pH 1.2-6.0) but gradually disintegrated in basic medium (pH > 7.0). Because of having such special pH sensitive characteristic, the co-polyester was tried to release the drug (diclofenac sodium) in simulated intestinal fluid. Diclofenac sodium from drug-loaded polymer matrix tablets was released at a steady rate (zero order) in simulated intestinal fluid for up to ten hours. Therefore, this co-polyester may be utilized as a drug carrier to release the medicine at a constant rate into the intestine. Nevertheless, toxicological and other pharmacological testing of this co-polyester must be carried out before a clinical trial.
Acknowledgement
The authors would like to thank the authority of Rajshahi University, Bangladesh, for providing facilities to carry out the research.
Funding Sources:
This research did not receive any specific grant from funding agencies in the public, commercial, or not-for-profit sectors.
Conflict of Interest:
The authors declare that there are no conflicts of interest regarding the publication of this manuscript.
Data Availability Statement
The manuscript incorporates all datasets produced or examined throughout this research study.
Ethics Statement
This research did not involve human participants, animal subjects, or any material that requires ethical approval.
Informed Consent Statement
This study did not involve human participants, and therefore, informed consent was not required.
Clinical Trial Registration
This study did not involve any clinical trial so clinical trial registration was not required.
Author Contributions
Dr. Abu Mahmud: Conceptualization, methodology, Data Collection, Analysis, Writing – Review & Editing.
Dr. Md. Abu Bakr: Conceptualization, methodology and supervision.
Dr. Jahanara Nasrin: TGA Data collection.
References
- Negut I., Bita B. Polymeric Micellar Systems—A Special Emphasis on “Smart” Drug Delivery, Pharmaceutics, 2023; 15(3): 976.
CrossRef
- Ozarde Y. S., Sarvi S., Polshettiwar S. A.,. Kuchekar B. S. Multiple-unit-pellet system (MUPS): a novel approach for drug delivery, Drug Invention Today 2012; 4: 603-609.
- Murthy P. N. V. N., Shafiullah D. and Datta M., Formulation, development and evaluation of controlled release matrix tablets of guaiphenesin and salbutamol sulphate, Der Pharmacia Letter, 2011; 3: 325-334.
- El-halim S. M. A., Amin M. M., Gazayerly O. N. E., Gawad N. A. A. E., Salbutamol sulphate controlled release hydrophilic matrix tablets, Journal of Pharmaceutical Sciences, 2011; 1: 194-200.
CrossRef
- Kumar S., Kumar A., Gupta V., Malodia and Rakha P., Oral Extended Release Drug Delivery System: A Promising Approach, Asian Journal of Research in Pharmaceutical Sciences, 2012; 2:101-106.
- Colombo P., Swelling controlled release in hydrogel matrices for oral route, Advanced Drug Delivery Reviews, 1993; 11: 37-57.
CrossRef
- Hu Y., Hu S., Zhang S. et al, A double-layer hydrogel based on alginate carboxymethyl cellulose and synthetic polymer as sustained drug delivery system. Sci Rep. 2021; 11: 9142.
CrossRef
- Engelberg I. and Kohn J., Physico-mechanical properties of degradable polymers used in medical applications: a comparative study, Biomaterials, 1991; 12: 292-304.
CrossRef
- Dai J., Liang M., Zhang Z., Bernaerts K. V., Zhang T., Synthesis and and characterization behavior of Poly (lactide-co-glycolide), Polymer, 2021; 235, 124-302.
CrossRef
- Rowe R. C., Sheskey P. J. and. Quinn M. E, Handbook of Pharmaceutical Excipients, Royal Pharmaceutical Society of Great Britain, 6th Edition, 2009; 82 and 593.
- Deore R. K., Kavitha K. and. Tamizhmani T. G, Preparation and evaluation of sustained release matrix tablets of tramadol hydrochloride using glyceryl palmitostearate, Tropical Journal of Pharmaceutical Research, 2010; 9: 275-281.
CrossRef
- Pramanick D. and Ray T. T. and Bakr M. A., Co-polyester of citric acid and 1,2,6-hexane triol as a matrix for controlled drug delivery, Journal of Polymer Meterials, 1996; 13: 173-178.
- Mahmud A. and Bakr M. A., Poly (maleic acid-co-propane-1,2-diol-co-adipic acid) for pH-triggered drug delivery, Reactive and Functional Polymer, 2015; 96: 21-24.
CrossRef
- Kumar R., Yakuba M. K., Anandjiwala R. D., Biodegradation of flux fiber reinforced poly lactic acid, eXPRESS Polymer Letters, 2010; 4(7), 423-430.
CrossRef
- Hoti G, Caldera F, Cecone C, Rubin Pedrazzo A, Anceschi A, Appleton SL, Khazaei Monfared Y, Trotta F. Effect of the Cross-Linking Density on the Swelling and Rheological Behavior of Ester-Bridged β-Cyclodextrin Nanosponges, Materials, 2021; 14(3):478.
CrossRef
- Wagner H. L., The Mark-Houwink-Sakurada equation for the viscosity of linear polyethylene, Journal of Physical and Chemical Reference data, 1985; 14, 611-617.
CrossRef
- Bakr M. A., Mahmud A., Sarker M., Islam M. A., Malic acid-Adipic Acid-Butane-1,4-Diol Co-polyester for Gastro-resistant Drug Delivery, Journal of Polymer Materials 2015; 32(1), 1-8.
- Kumer G. S., Kalpagam V. and Nandi U. S., Biodegradation of gelatin graft copolymers, Journal of Applied Polymer Science, 1985; 30: 915-924.
CrossRef
- Potts J. E. et al, Biodegradability of Plastics, United States Environmental Protection Agency, Contract No. CPE, 1972; 22: 70-124.
- Raju G., Hakim M. R., Haris M., Azura A. R., Amam E., Chitosan epoxidized natural rubber for sorption and biodegradability studies, ACS Omega, 2020; 5(44): 28760-28766.
CrossRef
- Mahmud A., Dev C., Meem M. T.,. Gafur M. A., Hoque M. A., Preparation and mechanical properties of epoxy/chitosan/silver nanocomposite, Green Materials, 2022; 10(3):110-118.
CrossRef
- Kelly L. S., Martin E. S., Karen E. T., Bioerodible polymers for delivery of macromolecules, Advanced Drug Delivery Reviews, 1990; 4(3); 343-357.
CrossRef
- Benhabbour S.R., Kovarova M., Jones C. et al.Ultra-long-acting tunable biodegradable and removable controlled release implants for drug delivery, Nat Commun, 2019; 10;
CrossRef
- Singh R.,Pal D., and Chattopadhyay S., Target-Specific Superparamagnetic Hydrogel with Excellent pH Sensitivity and Reversibility: A Promising Platform for Biomedical Applications, ACS Omega,2020; 5 (34): 21768-21780.
CrossRef
- Zhuo S., Zhang F., Yu J., Zhang X., Yang G., and Liu X., pH-Sensitive Biomaterials for Drug Delivery, molecules, 2020; 25: 5649.
CrossRef
- Liu L., Yao W., Rao Y., Lu X., & Gao J., pH-Responsive carriers for oral drug delivery: challenges and opportunities of current platforms, Drug Delivery, 2017, 24 (1): 569-581.
CrossRef
Abbreviations
PMPC: poly[(maleic acid)-co-(propane-1,2-diol)-ran-(citric acid)].

This work is licensed under a Creative Commons Attribution 4.0 International License.