Ramachandra Naik1,*
, V. Revathi1, S.C. Prashantha2, H. Nagabhushana3, K.M Girish4 and H.P Nagaswarupa5
1Department of Physics, New Horizon College of Engineering, Bangalore-560103, India
2Department of Science, East West Institute of Technology, Bangalore-560091, India.
3Department for advanced materials, Tumkur University, Tumkur-572103, India.
4Department of Physics, Dayananda Sagar Academy of Technology and Management, Bangalore, 560082, India.
5Department of Chemistry, Davanagere University, Davanagere, 577001, India.
Corresponding Author E mail: rcnaikphysics@gmail.com
DOI : http://dx.doi.org/10.13005/msri/170303
Article Publishing History
Article Received on : 28-October-2020
Article Accepted on : 4-Dec-2020
Article Published : 10 Dec 2020
Plagiarism Check: Yes
Reviewed by: Dr.Umesh Jagannath Tupe
Second Review by: Dr. Venkateswara Rao S
Final Approval by: Jit satyabrata
Article Metrics
ABSTRACT:
Low temperature solution combustion synthesized Cr3+(1- 4 mol%) doped Mg2SiO4 nanoparticles were analyzed by Powder X-ray Diffraction (PXRD), Fourier Transform Infra-Red (FTIR) spectroscopy, Cyclic voltammetry (CV) and Electrochemical Impedance Spectroscopy (EIS) techniques. PXRD profile shows the samples are crystalline.FTIR spectra show MgO6 octahedral and Si-O bending and stretching modes.It was observed that, CV show excellent semi rectangular shaped voltammograms due to the oxidation reduction reactions and the reversibility of the reaction which suits for electric double layer capacitance.Charge transfer resistance (Rct) was found to be 10 Ωindicates the better electron transfer from one phase to another.
KEYWORDS:
Combustion; Cyclic Voltammetry; Mg2SiO4; Nanoparticles
Copy the following to cite this article:
Naik R, Revathi V, Prashantha S. C, Nagabhushana H, Girish K. M, Nagaswarupa H. P. Cyclic Voltammetry and Electrochemical Impedance Spectroscopy Analysis of Cr3+ Doped Mg2SiO4 Nanoparticles for Supercapacitor Applications.Mat. Sci. Res. India; 17(3).
|
Copy the following to cite this URL:
Naik R, Revathi V, Prashantha S. C, Nagabhushana H, Girish K. M, Nagaswarupa H. P. Cyclic Voltammetry and Electrochemical Impedance Spectroscopy Analysis of Cr3+ Doped Mg2SiO4 Nanoparticles for Supercapacitor Applications.Mat. Sci. Res. India; 17(3). Available from: https://bit.ly/2VZeeob
|
Introduction
For as far back as not many decades a tremendous exertion has been centered around the creation of electrochemical capacitors or supercapacitors for higher force and vitality thickness than batteries and regular capacitors [1]. The carbon-based materials, progress metal oxides, and leading polymer are generally utilized as cathode material for an electrical double-layer capacitor (EDLC) and pseudocapacitors because of their high surface region and different change states. As of late, the progressively organized nanomaterials with a multi-scale association pulls in incredible consideration in light of its favorable circumstances, for example, [2, 3] amplifying the particular surface region and along these lines expanding its electrical twofold layer and pseudocapacitance conduct, making permeable inside spaces. Along these lines, the various leveled organized nanomaterial is a useful and perfect decision to be utilized as a terminal material for supercapacitors.
Supercapacitors are considered as an efficient power energy source and it very well maybe, for the most part, applied with different creation/blend techniques. It is outstanding amongst other fundamentally accessible sourcesof inefficient power energy innovation [4, 5]. The high surface zone (1344 m2g-1) and enormous pore volume (0.902 cm3g-1) of nitrogen-doped mesoporous/microporous carbon (NMMC) based cathode utilized in a supercapacitor, which has the conveyed capacitance estimation of 325 Fg-1 [6]. Zhang et al [7] utilized aqueous assisted technique for the blend of RGO/Ni3S2 on nickel froth anode, the predominantly performed apacitance estimation of 2188.8 Fg-1 at 2.9 Ag-1.
Silicates are an attractive class of materials among inorganic phosphors for a wide scope of uses because of their uncommon properties, for example, water, chemical resistance, and visible lighttransparency. Specifically, inorganic nanophosphors with the fuse of trivalent rare-earth cations uncovered significant iridescence impacts. Further, different opening and imperfections present in the host matrix result in different luminescence features [3]. Improved electrical, luminescent and optical properties of nano phosphors were brought about by the quantum size impact, which was created by an expansion in the bandgap because of a diminishing in the quantum permitted state and the high surface-to-volume ratio [10]. Among silicate family, the Mg2SiO4 have doped with rare earth and transition metal ions show some fascinating applications, for example, long-lasting phosphor, X-ray imaging, light-emitting diode (LED), environmental monitoring, and so forth [11–14].
Experimental
Mg2SiO4:Cr3+ (1- 4 mol%) nanoparticles have been prepared using solution combustion method as per the stoichiometry and by maintaining the fuel to oxidizer ratio unity. Raw materials taken are magnesium nitrate (Mg (NO3)2·6H2O (Sigma Aldrich, 99.99%), fumedsilica (SiO2(Sigma Aldrich, 99.99%), laboratory prepared oxalyldihydrazide (ODH; C2H6N4O2) fuel and chromiumnitrate (Cr(NO3)3: 99.9%, Sigma Aldrich). The mixture of these in 1-4 mol% of Cr3+is dissolved in a minimum quantity of doubled distilled water in a petri dish and mixed thoroughly using a magneticstirrer for about 5 min. The petri dish was introduced into the muffle furnace set at 350 oC. The solution turns out as a gel and then foam continues catching fire and finally forms a powder. The powder was ground and used for characterizations without calcination [15].
Results and Discussion
PXRD and FTIR analysis of as-prepared samples were carried out for structural confirmation. All the PXRD peaks were indexed and well-matched with JCPDS card No. 78-1371 with space group pbnm (No.62) and orthorhombic structure (Fig. 1). The lattice parameters and unit cell volume for (211) plane were estimated using the following relations and was found to be 2.471Å and 289.92×10-30 m3 respectively.
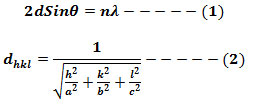
Figure 1: PXRD pattern of Mg2SiO4:Cr3+ (1-4 mol%) nanoparticle.
Cr3+ peaks were not observed which indicates that it is homogeneously mixed and effectively doped in the host lattice in Mg2+ sites [15]. The average crystallite size (D) was estimated from the line broadening in X-ray powder using Scherrer’s formula[39]
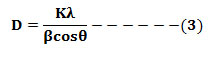
where, ‘K’; constant, ‘l’ ;wavelength of X-rays, and ‘b’; FWHM]was found to be in the range 25-35 nm. Using the FTIR analysis method, samples have been scanned by infrared light to determine bonds and confirm the prepared material (Fig. 2). The peaks at 425, 525, 625,685, 885, 1015, 1255 and 1405 cm−1 were assigned to MgO6 octahedral, Si–O (ν4), Si–O (bending), Mg–O, Si–O (ν3, stretching), (CO and Si–O), C–H and NO3 respectively, where ν3was asymmetric stretching and ν4 was asymmetric deformation vibrations [15].
Figure 2: FTIR pattern of Mg2SiO4:Cr3+ (1-4 mol%) nanoparticle.
CV and EIS were recorded at room temperature for all the prepared Mg2SiO4:Cr3+nanoparticles which shows remarkable electrochemical performance. CV analysis usually done to understand mass transportation and electron conduction. Shape of the curve is observed to be nearly rectangular, which is the characteristic of the electrical double layer capacitor. Increase of scan rates did not influence the mass transportation and electron conduction as observed in the diagram.Reversibility of the reaction, quantification of charge efficiency was understood with the help of CV analysis. By taking the difference between the oxidation potential (EO) and the reduction potential (ER) at the scan rate of 10 mV/s reversibility of the reaction was analyzed. Reversibility is high for smaller EO-ER. CV of Mg2SiO4: Cr3+ (1, 2, and 4mol %)recorded at scan rates from 0.01 V/s to 0.05 V/s were shown in Fig. (3-5).
Figure 3: CV pattern of Mg2SiO4:Cr3+ (1 mol%) nanoparticle
Figure 4: CV pattern of Mg2SiO4:Cr3+ (2mol%) nanoparticle.
Figure 5: CV pattern of Mg2SiO4:Cr3+ (4 mol%) nanoparticle.
Semi rectangular voltammograms are observed for all the samples. It was observed that, redox peak current increases with an increase in scan rate due to overpotential [16], andit was found maximum for 4 mol% doped sample. Redox peak shifted to lower potential due to the perfection in the reversibility of the electron transfer process and surface area of the modified electrode. At the extreme potential, current is found maximum because of electro-oxidation and reduction processes. The area under the 4 mol% curve is found to be maximum among all synthesized materials.This was mainly due to reduce in the ion diffusion path ensuring high utilization of surface pseudocapacitive reactions [17].
Figure 6: EIS pattern of Mg2SiO4:Cr3+ (1 mol%) nanoparticle.
Figure 7: EIS pattern of Mg2SiO4:Cr3+ (2mol%) nanoparticle.
Figure 8: EIS pattern of Mg2SiO4:Cr3+ (4mol%) nanoparticle.
Fig (6-8) shows the EIS (Nyquist plot) of Cr3+ (1, 2, and 4mol %) doped Mg2SiO4nanomaterials. The electrochemical impedance estimations were done in the recurrence go 1 Hz to 1 MHz with AC sufficiency of 5 mV at a consistent state. The semi-circle district in the Nyquist plot implies a high-frequency part and a linear area signifies a low-frequency part. The diameter of the semi-circle speaks to charge transfer resistance (Rct) and it was accounted for that littler the measurement of the circle, higher the charge transfer rate which offers a significant contribution to the improvement of photocatalytic action of the readied test. It was observed from the diagram that, Rct values for the Cr3+ (1, 2, and 4mol %) doped Mg2SiO4 nanomaterial was found to be 38 Ω, 11 Ω, and 10 Ω individually [18-20].
Conclusion
Low temperature (350 oC) initiatedsolution combustion technique used to prepare Cr3+ doped Mg2SiO4 results in as formed nanoparticles with no further calcination. The prepared samplesexhibitexcellent electrochemical performance in the wide potential range from 0 to – 0. 7 V. The Rct value for 4 mol% material is found to be the lowest among all presently synthesized nanomaterials (10Ω).These outcomes show that the sample is suitable for solid state electrochemical sensor development applications.
Acknowledgment
We thank the referees and the editor for their helpful comments and constructive suggestions that greatly improved the presentation of this paper.
Funding Source
This research received no specific grant from any funding agency.
Conflict of Interest
The authors have no conflicts of interest to disclose.
References
- Conway, B.E. Electrochemical Supercapacitors, Scientific Fundamentals and Technology Applications, Kluwer Academic/Plenum Press, New York, 1999.
- Wang X,Liu G,Lu GQ,Cheng, HM.; Int. J. Hyd.Ener., 35 (2010) 8199.
CrossRef
- Kim DW,Lee S,Suk Jung H,Young Kim J,Shin H,Hong KS,Int. J. Hyd. Ener., 15 (2007) 3137.
CrossRef
- BohknO,KowalJ,Sauer D.V, J. Power Sources, 172 (2007)468.
CrossRef
- Miller J.R,Electrochim. Acta, 52 (2006)1703.
CrossRef
- Song Y,Hu S,Dong D. X,Wang Y,Wang C,Wang C, and Xia Y,ElectrochimActa, 146 (2014) 485.
CrossRef
- Zhang Z,Zhao C,Min S, and Qian X,Electrochim. Acta, 144 (2014) 100.
CrossRef
- PrashanthaS.C,LakshminarasappaB.N,NagabhushanaB.M, J. Alloys. Compd. 509 (2011) 10185–10189.
CrossRef
- Cho S,Lee R,Lee H,Kim J,Moon C,Nam S,Park J, J. Sol–Gel Sci. Technol. 53 (2010) 171–175.
CrossRef
- Lee G.H, Kang S, J. Lumin. 131 (2011)2606–2611.
CrossRef
- He H,Fu R.L,Cao Y.G,Song X.F,Pan Z.W,Zhao X.R,Xiao Q.B,Li R. Opt. Mater.32 (2010) 632–636.
CrossRef
- TuD,LiangY,LiuR,ChengZ,Yang F,Yang W, J. Alloys. Compd. 509 (2011) 5596–5599.
CrossRef
- Ramachandra Naik, PrashanthaS.C, NagabhushanaH,Sharma S.C,Nagabhushana, B.M,Nagaswarupa, H.P,Premkumar, H.B,Sens. Act.B195 (2014)140– 149.
CrossRef
- Ramachandra Naik, PrashanthaS.C, Nagabhushana, H,Sharma S.C, Nagaswarupa, H.P,Girish K.M, J. Alloy. Compd682 (2016) 815-824.
CrossRef
- S.C. Prashantha, B.N. Lakshminarasappa, B.M. Nagabhushana, J. Alloy. compd. 509 (2011) 10185-10189.
CrossRef
- He Y,Chen W,Li X,Zhang Z,Fu J,Zhao C,XieE, ACS Nano, 7(2013)174-182.
CrossRef
- Preety Ahuja, Raj Kishore Sharma, Gurmeet Singh, J. Mater. Chem. A, 3(2015) 4931-4937.
CrossRef
- GurushanthaK,AnantharajuK.S,Sharma S.C,NagaswarupaH.P,PrashanthaS.C,Vishnu Mahesh K.R,RenukaL,VidyaY.S,NagabhushanaH, J. Alloy. Compd. 685(2016)761-773.
CrossRef
- P.R. Bueno, C. Gabrielli, H. Perrot, Electrochim. Acta 53 (2008) 5533–5539.
CrossRef
- Raghavendra S. Hebbar, Arun M Isloor, BalakrishnaPrabhu, Inamuddin, Abdullah M. Asiri and A.F. Ismail, Scientific Reports, 8(2018) 4665.

This work is licensed under a Creative Commons Attribution 4.0 International License.