Pradip Kumar Mondal and Deepak Chopra*
Department of Chemistry, Indian Institute of Science Education and Research, Bhopal, India
DOI : http://dx.doi.org/10.13005/msri/110106
Article Publishing History
Article Received on : 23 Aug 2014
Article Accepted on : 08 Sep 2014
Article Published : 09 Sep 2014
Plagiarism Check: Yes
Article Metrics
ABSTRACT:
Polymorphism is a widespread and commonly occurring phenomenon in fields of chemistry, biology and materials science. In recent years, the development of technology has lead to the development of different instrumentation tools(such as SXRD, PXRD, IR, NMR, AFM) which are employed for the characterization of different polymorphic materials (namely polymers, nano crystalline metal oxides and pharmaceutical drugs) which are of great importance because of their applications in the field of materials science.
KEYWORDS:
Polymorphism; widespread and commonly
Copy the following to cite this article:
Mondal P. K, Chopra D. Role of Polymorphism in Materials Science. Mat.Sci.Res.India;11(1)
|
Introduction
Polymorphism (Greek: poly = many, morph =form),which specifies the diversity existing in nature, is a term used in many disciplines [1]. The role of structural diversity emanates in almost every aspect in nature and the field of material sciences is no exception. Structural chemistry and crystal polymorphism is one such manifestation of that diversity. Polymorphism is a rapidly growing research area in material sciences because of innumerable application in fields of academic and commercial interest. Polymorphism plays an important role in the pharmaceutical, dyes, agrochemicals, and pigment industry, and most interestingly in the chocolate industry. In this short review, we intend to highlight the importance and characterization tools employed in the study of polymorphism in the solid state.
Single Crystal X-Ray Crystallography
Single crystal X-ray crystallographic techniqueis devoted towards the determination of the three dimensional atomic arrangement in a crystalline solid. This method is one of the most significant and unequivocal method for the characterisation of polymorphs. It isessential for the unique identification of individual polymorphic forms. The complete information on the bond lengths, bond angles, molecular conformation, and intermolecular interactions constitutes an indispensable component of crystal structure determination. In case of molecular crystals, the origin of polymorphism may be attributed towards differences in molecular conformation or arrangement of molecules in the solid state.One such case study has recently been reported in a biologically active compound, 6-(4-chlorophenyl)-5-(methoxycarbonyl)-4-methyl-2-(3-(trifluoromethylthio)phenylamino)-3,6-dihydropyrimidin-1-ium chloride which occurs as dimorphs [2]. The two forms have been characterized using single-crystal X-ray diffraction (XRD) [2]. Another interesting example is observed in the existence of four crystalline polymorphs of Fe2O3 [(a) α-Fe2O3 (b) β-Fe2O3 (c) γ-Fe2O3 (d) ε-Fe2O3] and has been characterized by single crystal X-ray diffraction. It is of interest to note the presence of significantly different structural properties (Fig-1) [3] in all the forms of this compound.
Powder X-ray Diffraction
Experimental powder X-ray diffraction (PXRD) is another definitive technique for the identification of polymorphs, particularly for powdered samples wherein crystallization techniques fail to give good quality single crystals. A new polymorph formation of NaSbO3was initially confirmed from PXRD [4]. Concomitant polymorphism in 3-fluoro-N-(3-fluorophenyl) benzamide has been confirmed by simulated powder XRD (Fig-2)[5].
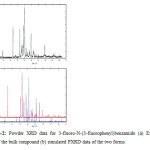 |
Figure2: Powder XRD data for 3-fluoro-N-(3-fluorophenyl)benzamide (a) Experimental data of the bulk compound (b) simulated PXRD data of the two forms.
Click here to View Figure
|
DSC, TGA and HSM
Thermal methods (DSC, TGA, and HSM) are based on the principle of phase change of a material by absorption or liberation of heat and it provides the relative stability of each polymorphic form. Differential scanning calorimetry (DSC) provides a quantitative measure of the relative stability of the two polymorphic forms, the energy involved in phase changes between them and the nature of the phase transition (monotropic or enantiotropic in nature). Thermogravimetric analysis (TGA) measures the change in the mass of a material as a function of temperature and it provides information on the loss of solvent from the solid, decomposition and sublimation nature of different polymorphic forms. Hot-stage microscopy (HSM), is a combination of microscopy and thermal analysis towards the study of polymorphism as a function of temperature and time. This visual examination gives information about the changes of compound at the melting point and other transformationsthat occur during the process of heating. The two polymorphic forms of the agrochemical, Metribuzin have been characterized by the technique of differential thermal analysis (DTA) and exhibits different melting temperatures for the needles and the plate form (Fig-3) [6].
IR and Raman spectroscopy
Infrared and Raman spectroscopy gives information on the vibrational modes in a crystalline solid and these techniques follow different selection rules. IR is an absorption phenomenon and IR radiation is absorbed by a polar group of a molecule. On the contrary, Raman spectroscopy is a scattering phenomenon and it has an effect on the symmetric vibrations and nonpolar groups of a molecule. The magnitude of the IR peaks for the presence of different functional groups can be altered to different magnitudes in the case of polymorphs. Three polymorphic form (β-, γ-, δ-form) of poly(3-hydroxypropionate) has been investigated by variable-temperature FTIR spectroscopy. The β- and γ-form exhibit similar FTIR spectra in the fingerprint region, indicating that they adopt a similar conformation. But δ-form shows a very different FTIR spectrum due to the different chain conformation (Fig-4) [7].
![Figure-4: Temperature-dependent FTIR spectra in the fingerprint region for (a) β–form, (b) γ-form, (c) δ–form crystals of poly(3-hydroxypropionate) [7].](http://www.materialsciencejournal.org/wp-content/uploads/2014/09/Vol11_No1_Role_Pradi_fig4-150x150.jpg) |
Figure4: Temperature-dependent FTIR spectra in the fingerprint region for (a) β–form, (b) γ-form, (c) δ–form crystals of poly(3-hydroxypropionate) [7].
Click here to View Figure
|
Solid state NMR spectroscopy:
Solid state NMR spectroscopy gives information on the environment of the individual atoms which is different for polymorphs and it is not limited to a single nucleus. It can be applied for molecules containing1H, 13C, 15N, and31P chemical nuclei in different environments in polymorphs. For polymorphs, the differences in molecular conformation, changes in crystal packing, and the presence of different intermolecular interactions may be reflected in changes in the solid state NMR. Solid-state NMR techniques can be used for a full structural characterization of polymorphs. 31P{1H} cross-polarization magic angle spinning (CPMAS) NMR spectra of P,P-[3]ferrocenophanes [8] for a series of preparations using different crystallization conditions, indicating that polymorphs A and B with different environment and the formation of the second polymorph B is governed by extended storage of polymorph A at room temperature. 31P{1H} (CPMAS) NMR spectra (Fig-5) which indicate third polymorphs C also and this is present in minor amounts in all samples containing polymorph A.
![Figure-531P{1H} (CPMAS) NMR spectra of a series of samples. (a1) Solvent (dichloromethane) removed by vacuum, (a2) crystallized overnight, (a3) crystallized over two days, (a4) crystallized over five days. (b) 31P{1H} (CPMAS) NMR spectra showing a sample of polymorph B exposed to CH2Cl2 vapour at room temperature in a desiccator for 48h. (c) 31P{1H} (CPMAS) NMR spectra of the optically pure compound [8].](http://www.materialsciencejournal.org/wp-content/uploads/2014/09/Vol11_No1_Role_Pradi_fig5-150x150.jpg) |
Figur-531: P{1H} (CPMAS) NMR spectra of a series of samples. (a1) Solvent (dichloromethane) removed by vacuum, (a2) crystallized overnight, (a3) crystallized over two days, (a4) crystallized over five days. (b) 31P{1H} (CPMAS) NMR spectra showing a sample of polymorph B exposed to CH2Cl2 vapour at room temperature in a desiccator for 48h. (c) 31P{1H} (CPMAS) NMR spectra of the optically pure compound [8].
Click here to View Figure
|
Solid state NMR spectroscopy
Solid state NMR spectroscopy gives information on the environment of the individual atoms which is different for polymorphs and it is not limited to a single nucleus. It can be applied for molecules containing1H, 13C, 15N, and31P chemical nuclei in different environments in polymorphs. For polymorphs, the differences in molecular conformation, changes in crystal packing, and the presence of different intermolecular interactions may be reflected in changes in the solid state NMR. Solid-state NMR techniques can be used for a full structural characterization of polymorphs. 31P{1H} cross-polarization magic angle spinning (CPMAS) NMR spectra of P,P-[3]ferrocenophanes [8] for a series of preparations using different crystallization conditions, indicating that polymorphs A and B with different environment and the formation of the second polymorph B is governed by extended storage of polymorph A at room temperature. 31P{1H} (CPMAS) NMR spectra (Fig-5) which indicate third polymorphs C also and this is present in minor amounts in all samples containing polymorph A.
|
Figure6: SEM image of sexithiophene films (a) at 77K (small grains of 10-30 nm), (b) Room temperature (isotropic grains with a diameter of 50nm), (c) at 190˚C (elongated grains of 30 x 200 nm2), and (d) at 260˚C (50 nm wide small plate connected with each other) [9].
Click here to View Figure
|
Atomic Force Microscopy
Atomic force microscopy gives the structure of surfaces of the crystallizing material. This can be used to study the nucleation and selective control of the growth of a crystal. The stereo irregular sample isotactic polypropylene (iPP) [10] crystallized in different polymorphic forms (γ, α and mesophase) which shows elastic properties. Fig-7 shows the AFM image for the different phases (A-C) of a sample of iPP(5) of differently crystallized polymorphic forms.
![Figure-7: AFM phase of sample of iPP(5) (A) thin and elongated morphology of the γ form (B) nodules of the mesomorphic form (C) nodules of the α form [10].](http://www.materialsciencejournal.org/wp-content/uploads/2014/09/Vol11_No1_Role_Pradi_fig7-150x150.jpg) |
Figure7: AFM phase of sample of iPP(5) (A) thin and elongated morphology of the γ form (B) nodules of the mesomorphic form (C) nodules of the α form [10].
Click here to View Figure
|
Polymorphism in Polymer Science
Polymorphism in materials science represents the existence of more than one crystalline structure, for example different crystal packing with the same chemical composition. The class of polymorphic polymer crystals may arise from different conformation or the different packing modes of a molecular chain in the unit cell [7]. Three polymorphic forms of iPP (α-, β-, and γ-form) is an example of a packing polymer with the same threefold helical conformation [7, 10]. A stable polymorph is thermodynamically controlled while the less stable polymorph usually considered to be metastable is due to kinetic effects.
Polymorphic behaviour can be controlled by many factors such as miscible material, molecular weight, melting conditions, nucleation of polymer etc. One of the important factors is the presence of nucleating agents which can change the crystalline structure of a polymorphic polymer and these are widely used in industries. Different type of polymorphic forms of iPP (α-, β-, and γ-form) have been controlled by nucleating agents. Molecular weight and the chain micro structures (e.g., stereo regularity, region regularity, region defects, and stereo defects) affect the crystalline structures.
The bacterial poly(3-hydroxybutyrate) can crystallize in two forms (α and β) and α-form is easy to produce by normal conditions such as melt or solution crystallization. The α-form is characterized by two anti parallel chains packed in an orthorhombic unit cell (the space group is P212121). While the β-form crystal of poly(3-hydroxybutyrate) were first observed by XRD analysis with a hexagonal unit cell [7,11]. A typical X-ray diffraction pattern (XRD) of the two forms (α and β) is shown (Fig-8).
![Figure-8: Molecular structure of poly(3-hydroxybutyrate) with two polymorphic forms, 21 helix for the α–form and planar zigzag conformations for β-form. (A) α–form (B) both α– and β-form. The arrow indicates a typical equatorial reflection of the β-form of poly(3-hydroxybutyrate) [11].](http://www.materialsciencejournal.org/wp-content/uploads/2014/09/Vol11_No1_Role_Pradi_fig8-150x150.jpg) |
Figure8: Molecular structure of poly(3-hydroxybutyrate) with two polymorphic forms, 21 helix for the α–form and planar zigzag conformations for β-form. (A) α–form (B) both α– and β-form. The arrow indicates a typical equatorial reflection of the β-form of poly(3-hydroxybutyrate) [11].
Click here to View Figure
|
Polymorphism in nanocrystalline metal oxides
Polymorphism of metal oxides in bulk has been widely studied and specifically with respect to the presence of nanocrystalline metal oxides. Polymorphism is found in different types of metal oxide, such as TiO2, CrO2, SnO2, Cr2O3, Fe2O3, and Al2O3. Structural changes occur due to polymorphic transitions under different conditions of temperature and pressure in metal oxides. Fe2O3 exists in four different polymorphic forms [(a) α-Fe2O3 (b) β-Fe2O3 (c) γ-Fe2O3 (d) ε-Fe2O3], where α-Fe2O3 form is the most stable in comparison to the other three metastable forms (Fig-1)[3]. Al2O3exhibits several polymorphic form such as α-, γ-, δ-, κ-, ρ-form [12, 13].
TiO2 also exists in several polymorphic form, such as the thermodynamically stable rutile [tetragonal; a = 4.5937Å, c = 2.9587Å, space group P42/mnm), kinetically stable anatase [tetragonal; a = 3.7845Å, c = 9.5143Å, space group I42/amd) and broookite [orthorhombic; a = 5.4558Å, b = 9.1819Å, c = 5.1429Å space group Pcab) [12, 14]. Structural changes in TiO2 are significantly controlled by the pH of the reaction medium, which includes using TiCl4 and KOH solution. The pure rutile phase is produced mainly in highly acidic medium or the concentrated solution of TiCl4 and the presence of a basic medium leads to preferred formation of the anatase phase. At pH = 7.1, the pure anatase phase is formed and at pH = 3.4, the product was anatase with some brookite phase. When pH = 1.0, a small proportion of the rutile phase is also present. The product morphologies are shown in Fig-9 [15].
![Figure-9: TEM micrographs of the products prepared at different pH: (a) pH = 7.1, (b) pH = 3.4, (c) pH = 0.0, and at different concentration of TiCl4 (d) 0.44 mol dm-3, (e) 0.53 mol dm-3, (f) 1.40 mol dm-3 [15].](http://www.materialsciencejournal.org/wp-content/uploads/2014/09/Vol11_No1_Role_Pradi_fig9-150x150.jpg) |
Figure9: TEM micrographs of the products prepared at different pH: (a) pH = 7.1, (b) pH = 3.4, (c) pH = 0.0, and at different concentration of TiCl4 (d) 0.44 mol dm-3, (e) 0.53 mol dm-3, (f) 1.40 mol dm-3 [15].
Click here to View Figure
|
Drug Polymorphism
Polymorphism is a very common phenomenon with more than one third of the drugs in the pharmaceutical industry is found to exhibit solid state diversity. This can influence every aspect of the solid state properties of a pharmaceutical drug. The main factors which are affected are the dissolution properties and the solubility of the crystalline form for the formation of a drug product. Such studies also have direct relevance with bioavailability. The development of a pharmaceutical polymorph can be a long, arduous and expensive process and this involves preparation, identification, characterization, phase purity and properties of the formed crystals. In Table 1, we highlight the occurrence of the number of polymorphic forms in some of the most important drugs in the pharmaceutical industry [16].
Table 1
Drug
|
No of polymorphs
|
Chloramphenicol Palmitate
|
2
|
Carbamazepine
|
4
|
Phenyl butazane
|
5
|
Sulfapyridine
|
7
|
Spironolactone
|
6
|
Terazosin HCL
|
3
|
2-Amino 5-Nitropyridine
|
3
|
Eztrene
|
3
|
Ampicillin
|
3
|
Estradiol
|
4
|
Arteether
|
6
|
Benzimidazole
|
3
|
Sitafloxacin
|
2
|
Conclusions
The area of polymorphism is rich, exciting and challenging. Although it involves serendipity, yet the discovery, isolation and characterization of the different solid state phases of different materials is of significance. This is because of its direct relevance in the properties of materials. The future is expected to witness more interesting discoveries related to prevalence of polymorphism in nature.
References
- Bernstein, J. Polymorphism in Molecular Crystals; Oxford University Press: Oxford, Great Britain, 2002.
- Panini P., Venugopala K. N., Odhav B. and Chopra D., Acta Cryst. B70, (2014) 681-696.
CrossRef
- Zboril R., Mashlan M. and Petridis D., Chem. Mater. 14, (2002) 969-982.
CrossRef
- Mizoguchi H., Woodward P. M., Byeon S. –H., Parise J. B. J. Am. Chem. Soc. 126, (2004) 3175-3184.
CrossRef
- Chopra D. and Guru Row T. N. Cryst. Growth & Des., 8, (2008) 848-854.
CrossRef
- Chopra D., Mohan T. P., Rao K. S., and Guru Row T. N. CrystEngComm, 7(62), (2005) 374-379.
CrossRef
- Zhu B., Kai W., Pan P., Yazawa K., Nishida H., Sakurai M. and Inoue Y. J. Phys. Chem. B, 112, (2008) 9684-9692.
CrossRef
- Wiegand T., Ludeker D., Brunklaus G., Bussmann K., Kehr G., Erker G., Eckert H., Dalton Trans., 43, (2014) 12639-12647.
CrossRef
- Servet B., Horowitz G., Ries S., Lagorsse O., Alnot P., Yassar A., Deloffre F., Srivastava P., Hajlaoui R., Lang P. and Gamier F., Chem. Mater., 6, (1994) 1809-1815.
CrossRef
- Rosa C. D., Auriemma F., Girolamo R. D., Ballesteros O. R., Pepe M., Tarallo O., and Malafronte A., Macromolecules 2013, 46, 5202-5214.
CrossRef
- Iwata T., Fujita M., Aoyagi Y., Doi Y. and Fujisawa T., Biomacromolecules, 6, (2005) 1803-1809.
CrossRef
- Sood S., Gouma P., Nanomaterials and Energy, 2, Issue NME2, (2013) 82-96.
- Tsukada T., Segawa H., Yasumorib A. and Okada K., J Mater. Chem., 9, (1999) 549-553.
CrossRef
- Suresh C., Biju V., Mukundar P. and Warrier K. G. K., Polyhedron, 06, (1998) 2020-2024.
- Cheng H., Ma J., Zhao Z., and Qi L., Chem. Mater. 7, (1995) 663-671.
CrossRef
- Pangakar P. A., Tayade A. M., Uttarwar S. G. and Wanare R. S., International Journal of Pharmarcy & Technology, 5, (2013) 2374-2402.

This work is licensed under a Creative Commons Attribution 4.0 International License.