Adsorption of Acid Dye by Activated Carbon from Agricultural Solid Waste Leucona Leucocephala Seed Shell Waste: Kinetics, Equilibrium and Isotherm Study
Babu Rajendran A1, Kalaiselvan S2* and Padmavathi R3
1Department of Chemistry, Government Higher Secondary School, Dharapuram, Tirupur, Tamilnadu, India.
2Department of Chemistry, SNS College of Technology, Coimbatore, Tamilnadu, India
3Department of Chemistry, M.Kumarasamy College of Engineering, Karur, Tamilnadu, India.
Corresponding Author E-mail: kalaichem82@gmail.com
DOI : http://dx.doi.org/10.13005/msri/170307
Article Publishing History
Article Received on : 22-April-2020
Article Accepted on : 23-Dec-2020
Article Published : 25 Dec 2020
Plagiarism Check: Yes
Reviewed by: Dr. Pamarti Viswanath
Second Review by: Dr. Vishnu Adole
Final Approval by: Dr. K. M. Garadkar
Article Metrics
ABSTRACT:
Activated carbons from Leucaena leucocephala Seed Shell left over were generated accompanied by 10% phosphoric acid solution in the N2 atmosphere by chemical activation and their characteristics were investigated. SEM, FTIR and XRD analysis were used to analyse the characteristics of the triggered preparations. Isothermal models, namely the Freundlich and Langmuir representations, were the equilibrium studies carried out.. The equilibrium adsorption result was better suited to the isotherm model of Langmuir and its maximum monolayer adsorption potential for acid blue was 70.67 mg/g. Adsorption kinetics studies have shown that the best fit is provided by the pseudo second-order dynamic model. An intraparticle dispersion model demonstrated that the dissemination of the intraparticle was not a step of rate control. Studies of thermodynamics have shown that the sorption mechanism is spontaneous and exothermic in nature. The findings suggest that the seed shells of Leucaena leucocephala may be used as a possible adsorbent for Acid blue1 adsorption.
KEYWORDS:
Acid Blue1; Isotherm Models; Kinetic Models; Leucaena Leucocephala Activated Carbon; Thermodynamic Studies
Copy the following to cite this article:
Rajendran A. B, Kalaiselvan S, Padmavathi R. Adsorption of Acid Dye by Activated Carbon from Agricultural Solid Waste Leucona Leucocephala Seed Shell Waste: Kinetics, Equilibrium and Isotherm Study. Mat. Sci. Res. India; 17(3).
|
Copy the following to cite this URL:
Rajendran A. B, Kalaiselvan S, Padmavathi R. Adsorption of Acid Dye by Activated Carbon from Agricultural Solid Waste Leucona Leucocephala Seed Shell Waste: Kinetics, Equilibrium and Isotherm Study. Mat. Sci. Res. India; 17(3). Available from: https://bit.ly/3pqPphw
|
Introduction
Activated carbon is among the finest extensively acknowledged adsorbents on account of its high adsorptive limit for that reason, it has been usually exploited as adsorbent [1,2] and in catalysis [3,4] or Separating Techniques [5,6]. Thus, the significance for activated carbon is escalating. In spite of, Actived carbon is pricey which confine its substantial choice application. from this day forward, minimal effort woodland and rural squanders are viewed as promising adsorbents for adsorption applications. In addition, they are less expensive and promptly reachable materials. Lately, a ton of examination has been accounted for on enacted carbons from farming squanders, for example, [7], sago squander [ 8], pine saw dust [ 9], sugar stick dust [10], coir substance [11], oil palm squander [12], coconut shell squander [ 13], tropical wood [14], saw dust [15], pinus pinaster bark [16], corncob [17], eucalyptus bark [18], pistachio shells [19], babool wood [20], Feronia limonia [21], Leucaena leucocephala seed shell [22], Pongamia pinnata [23], Jatropha curcas [24]. There were also few reports on adsorbents employed in water purification [25,26], Poison Removal [27] Heavy metals Removal [28]. This examination uncovers that the enacted carbon arranged from Leucaena leucocephala seed shell squander as a farming adsorbent and the adsorption of corrosive blue1 was researched.
Materials and Methods
From the town of Tirupur, Leucaena leucocephala seed shell left over is gathered and dried and broken into little pieces. The pieces were then impregnated for 2 hours with a 10 % phosphoric acid solution and immersed for 24 hours in a same solution. The excess solution was decanted and dried in the air. In the muffle furnace, the material was then carbonized at 120-130 °C. The dried material was powdered and triggered for a period of 60 minutes in the muffle furnace at 800 C. The sample was then washed with a high volume of water to extract residual acid, and the dried and powdered sample was placed in sealed flasks. Scanning Electron Microscopy S EM characterised the prepared activated carbon morphological sample. FTIR analysis determined the character of functional groups.
Dye Adsorption Measurements
Of all the reagents used, the AR grade was (E-merk). Different Acid Blue1 dye solution concentrations were prepared with distilled water. Batch mode adsorption experiments were conducted in a thermostated water bath with the addition of 50 mg of adsorbent and 50 mL of dye solution shaker with certain concentrations, varying pH and temperatures. The samples were extracted at fixed time intervals from the shaker and the solutions were isolated by centrifugation from the adsorbent. Before and after adsorption, the dye concentrations in the solutions were measured using the Elico UV visible spectro photo meter. Using a 0.1N NaOH or HCl solution, the Ph of the solution for the dye was changed. The adsorption experiments were performed at various temperatures. The adsorbed dye quantity q (mg/g) and the efficiency of adsorption is determined as follows: 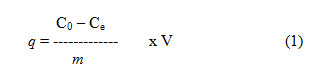
Adsorption Efficiency (%) =
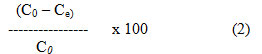
where, Co = original dye concentration (mg/L)
Ce = equilibrium concentration (mg/L)
V = volume of solution (ml)
m = mass of adsorbent (g)
C = remaining dye concentration (mg/L)
q = quantity of dye adsorbed (mg/g)
m = mass of adsorbent (g)
C = remaining dye concentration (mg/L)
q = amount of dye adsorbed (mg/g)
Results and Discussion
SEM analysis
Scanning electron microscope (SEM) image of the carbon sample prepared in Figure 1. The SEM images clearly showed that the sample’s outer surfaces were rough and Pores of various sizes and shapes were included, such as the honeycomb structure. There were tiny elongated pores on the surface, which are consistent with the well-developed porosity. The micrographs showed that the cavities on the carbon sample surfaces resulted from the evaporation of H3PO4 at moderate temperatures during activation, creating an empty room. The molecules of the chemical impregnating agent diffuse through the texture of the lignocellulosic material during impregnation.
Figure 1: SEM image for LSAC
Chemical Surface Characterization
Surface functional groups contained by activated carbon contribute considerably to its adsorption capability, such as ion exchangers, adsorbents, catalysts, and catalyst supports. The Fourier Transform Infrared Spectroscopy (FTIR) spectrum of the precursor and LSAC is presented in figure 2. At 2800-3000 cm-1, the precursor had a peak that was ascribed to the C-H, but the LSAC spectrum was reduced. noteworthy differences happened in the 1400-1700 cm-1 cm bands. For the spectrum of the precursor, the band at 1616 cm−1 is allocated to C=O. Changes in the FT-IR spectrum of LSAC have provided signs of the creation of structures comprising many carbon-carbon bonds, as well as the elimination of the originally present oxygen and hydrogen atoms.
Figure 2: FTIR Spectroscopy for LSAC
XRD analysis
Figure 3 confirms that the activated carbon material derived from Leuceana leucocephala is characterised by a large amorphous phase content in the carbon surface structure.
Figure 3: XRD pattern for LSAC
Adsorption Kinetics
For Acid blue 1 at pH 6.5 up to 240 min, the kinetic of adsorption was studied by varying the initial dye concentration to facilitate investigating the behaviour of acid blue1 adsorption method on LSAC, three kinetic models were applied to adsorption kinetic data. Lagergren offers a basic kinetic study of adsorption, pseudo first-order kinetics and its incorporated shape. [29].

where kL is the pseudo first order rate constant.
A plot of log (qe-qt) vs time for acid blue 1adsorption onto LSAC facilitates computation of the rate constant k1 and qe from the slope and intercept of the plot outline (4)
Figure 4: Effect of pseudo first order plot for the adsorption of acid blue 1onto LSAC
To illustrate dye adsorption, the tailored pseudo second order kinetic equation [30] is articulated as 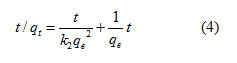
where, k2 is the pseudo second order rate constant. A plot of t/qt Vs t for acid blue1 adsorption on LSAC is revealed in figure (5), which facilitates computation of the rate constant k2 which is used to estimate the original
sorption rate h as follows

Figure 5: Effect of pseudo second order scheme for the adsorption of acid blue1 onto LSAC
Figure 6: Effect of intraparticle diffusion plot for the adsorption of acid orange 7 onto LSAC
The solute relocate is typically marked by exterior mass transfer or intra-particle dissemination or both for a solid-liquid adsorption method. To describe the mechanism involved in the adsorption process, the intra-particle diffusion model projected by Weber and Morris [31] was utilized.
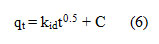
where kid (mg/g min0.5) is the rate constant of the intra-particle dissemination representation and C (mg/g) replicates the boundary layer outcome. The kid and C can be established from the slope and intercept of the plot qt Vs t0.5 and is shown in Figure (6) for acid blue 1adsorption. As the dye concentration increased, the constant pseudo first order and second order rate decreased. According to the model of intra particle diffusion, if intra particle diffusion is concerned in the adsorption method, the plot of uptake should be linear, and if the line passes through the origin, then the rate control step is intra particle diffusion. It is an indicator of boundary layer control when the plot does not move through the source. This reveals that intra-particle dissemination only is not the rate-limiting stage, but the rate of adsorption also regulated by other kinetic models, all of which can work simultaneously.
Table 1: Kinetic factors for the adsorption of acid blue1 on LSAC
Model
|
Parameters
|
Concentration (in mg/L)
|
20
|
40
|
60
|
Pseudo first order
|
K1
|
0.0137
|
0.0096
|
0.0078
|
R2
|
0.9301
|
0.9688
|
0.9715
|
Pseudo second order
|
K2
|
0.0323
|
0.00587
|
0.0026
|
h
|
3.7786
|
2.9923
|
2.5508
|
qe
|
10.8145
|
22.5744
|
31.1593
|
R2
|
0.9987
|
0.9911
|
0.9921
|
Weber Morris
|
Kid
|
0.5508
|
1.1662
|
1.5793
|
R2
|
1.000
|
0.9981
|
0.9772
|
Adsorption Isotherms
In this work, the Langmuir and Freundlich models were fitted with adsorption isothermal data of acid blue 1dye for variation in the most commonly used activated carbon (LSAC) dose. The isothermal fits were compared using the correlation coefficient (R2) and were listed in the table (1 & 2). The Langmuir model [32] assumes that a solid surface carries a small number of sites characterised by equivalent adsorption energy, irrespective of the degree of coverage and thus implying an equal amount of adsorption energy.
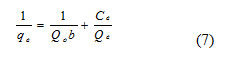
where, Qo is the highest adsorption capability, b is the Langmuir binding energy coefficient. The b and Qo can be intended from the intercept and slope of the linear system of Ce/qe against Ce as revealed in figure (7).
Figure 7 : Effect of langmuir plot for the adsorption of acid blue 1onto LSAC
Figure 8: Effect of Freundlich plot for the adsorption of acid blue 1onto LSAC
The Langmuir adsorption isotherm is clearly more fitting to characterise the adsorption equilibrium (R2 > 0.99). Monolayer coverage of acid blue 1onto LSAC particles with maximum adsorption potential of 66.21, 70.67 and 66.91 mg/g at 30, 45 and 60 oC was therefore obtained. Because of the available sites present in the carbon surface, the adsorption potential was increased by 30 and 45oC, then decreased by 60 oC because the sites were not available or desorption would take place. As the temperature rose, the KL values decreased. The freundlich isotherm [33] is one of the most usually utilized isotherms for the depiction of multi-site adsorption and is an empirical condition.
The linear form as follows:

Where Kf and n are the constants of the Freundlich isotherm indicating the respective adsorption potential and adsorption strength. It is possible to determine Kf and n from the intercept and slope of the linear plot of log qe against log Ce (9). The value of n suggests that acid blue 1onto LSAC has beneficial adsorption.
Figure 9: Effect of Freundlich plot for the adsorption of acid blue1 onto LSAC
Table 2: Isotherm parameters for the adsorption of acid blue1 onto LSAC
Temp
|
Langmuir Isotherm
|
Freundlich Isotherm
|
Q0(mg/g)
|
KL (L/mg)
|
R2
|
Kf
|
n
|
R2
|
30 °C
|
66.2177
|
5.7443
|
0.9912
|
7.8161
|
1.9375
|
0.9768
|
45 °C
|
70.6773
|
4.3305
|
0.9886
|
6.0587
|
1.7965
|
0.9764
|
60 °C
|
66.9125
|
4.1225
|
0.9911
|
6.5898
|
2.102
|
0.9861
|
Thermodynamic parameters for the adsorption
The thermodynamic factors such as free energy (ΔG), enthalpy (ΔH), and entropy (ΔS) give a depiction of adsorption performance correlated to temperature. Parameter can be calculated from
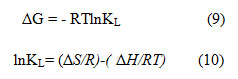
R value is the constant (8.314 J / (mol.K), where KL (L/mol) is from the Langmuir equation, and T (K) is the temperature in Kelvin. As can be observed from Table 3, at all temperatures measured, the estimations of ΔG were reliably negative, which uncovered that the adsorption cycle was viable and spontaneous process in nature. In addition, the unconditional values of ΔG put forward that the phase of sorption is highly desirable at low temperatures. The negative value of ΔH was representative of the adsorption process’s exothermic existence. In the solid-solute adsorption systems, the negative value of ΔS implied diminished uncertainty, which designated that the increase in entropy produced by the displaced water molecules is lower than that lost by the acid blue 1 molecules.
Table 3: Thermodynamic parameters for the adsorption of acid blue 1 onto LSAC
ΔH
(kJ/mol)
|
ΔS
(J/(molK))
|
ΔG (kJ/mol)
|
303K
|
318K
|
333K
|
-52.9775
|
-155.0539
|
-5098.7470
|
-1280.8649
|
-4617.6112
|
Conclusions
In this analysis, the low energy consumption of the Leucaena leucocephala seed shell was modified using the H3PO4 process. The kinetic adsorption fit the pseudo-second-request model well. The intraparticle dissemination model outcomes show that intraparticle dispersion was not by any means the only advance deciding rate. The Langmuir isotherm model was found to yield the best equilibrium outcome for corrosive blue with a most intense monolayer adsorption of 70.67 mg/g. Investigations of thermodynamics have shown the natural and exothermic presence of the component of sorption process.
Acknowledgment
The authors acknowledge Karunya University-Coimbatore for access to Electron Microscopes and Bharathiar University-Coimbatore for Technical support.
Conflict of interest
The authors declare that there is no conflict of interests regarding the publication of this article.
Funding Source
This research received no specific grant from any funding agency.
References
- Y.T. Hung, H.H. Lo, L.K. Wang, J.R. Taricska, K.H. Li, Granular activated carbon adsorption, in: L.K. Wang, Y.T. Hung, N.K. Shammas (Eds.), Handbook of Environmental Engineering, vol. 3, Humana Press, Totowa, New Jersey, 2005, pp.573–634.
CrossRef
- R. Taylor, Carbon blacks: production, properties and applications, in: H. Marsh, E.A. Heintz, F. Rodriguez-Reinoso (Eds.), Introduction to Carbon Technologies, Universidad de Alicante, Secretariado de Publicaciones, Alicante, 1997, pp. 167–210.
- J. Lee, J. Kim, T. Hyeon, Recent progress in the synthesis of porous carbon materials, Adv. Mater. 18 (2006) 2073–2094.
CrossRef
- W.M.T.M. Reimerink, The use of activated carbon as catalyst and catalyst carrier in industrial applications, Stud. Surf. Sci. Catal. 120A (1999) 751–769.
CrossRef
- F. Rodriguez-Reinoso, Production and application of activated carbon, in: F. Schuth, K.S.W. Sing, J. Weitkamp (Eds.), Handbook of Porous Solids, Wiley–VCH, Weinheim, Germany, 2002, pp. 1766–1827.
- A. Dabrowski, Adsorption from theory to practice, Adv. Colloid Interface Sci. 93 (2001) 135–224.
CrossRef
- K. Kadirvelu, M. Kavipriya, C. Karthika, M. Radhika, N, Vennilamani and S. Pattabhi, “Utilization of Various Agricultural wastes for Activated carbon Preparation and Application for the Removal of dyes and Metal ions from Aqueous solution” Biores. Technol., vol. 87, pp. 129- 132, 2003.
CrossRef
- T. Vaughan, C.E. Seo and W.E. Marshall, “Removal of Selected metal ions from Aqueous solution using Modified corncobs”, Biores. Technol., vol. 78, pp. 133-139, 2001.
CrossRef
- Satish Manocha, B. Vanraj Chauhan, and L.M. Manocha, “Porosity development on Activation of Char from dry and Wet babool wood”, Carbon Science, vol. 3, no. 3, pp. 133-141, 2002.
CrossRef
- R. Akkaya and U. Ulusoy, “Adsorptive features of chitosan entrapped in polyacrylamide hydrogel for Pb2+, UO22+ and Th4+”, J. Hazard. Mater., vol. 151, pp. 380-388, 2008.
CrossRef
- C. Kannan, B. Sivakumar, P. Nithya and S. Karthikeyan, “Thermodynamic, Kinetic and Equilibrium Studies on the sorption of reactive blue-2 from aqueous solution in the Balsamodendron Caudatum Wood waste”, Ind. J. Sci., vol. 4, no. 11, pp. 45, 2013.
CrossRef
- K, Kathirvelu, C. Karthika, N. Vennilamani and S. Pattabhi, “Activated carbon from Industrial solid waste as an Adsorbent for the Removal of Rhodamine-B from Aqueous solution: Kinetic and Equilibrium studies”, Chemosphere, vol. 60, pp. 1009, 2005.
CrossRef
- M. Ozacar and A.I. Sengal, “Adsorption of Metal complex dyes from Aquoues solutions by Pine Sawdust”,Bioresource Technol., vol. 96, pp. 791, 2005.
CrossRef
- M. K. Khattri Vand Singh, “Colour removal from Dye Wastewater using sugar cane dust as an adsorbent”, Adsorption Sci.Technol., vol. 17, pp. 269, 1999.
- K. Kairivelu, K. Thamaraiselvi and C. Namasivayam, “Adsorption of Nickel(II) from Aqueous solution onto Activated carbon prepared from Coirpith”, Sep. and Pur. Technol., vol. 24, pp. 497, 2001.
CrossRef
- C.A. Lua and J. Guo, “Preparation and Characterisation of Chars from Oil palm waste”, Carbon, vol. 36, pp. 1663–1670, 1998.
- C.J. Kirubakaran and S.K. Krishnaiah Seshadri, “Experimental study of the production of Activated carbon from Coconut shells in A Fluidised Bed Reactor”, Ind. Eng. Chem. Res., vol. 27, pp. 2411–2416, 1991.
CrossRef
- K. Maniatis and M. Nurmala, “Activated carbon production from Biomass”, Biomass Energy Ind. Environ., vol. 274, pp. 1034, 1992.
CrossRef
- V.C. Taty-Costodes, H. Fauduet, C. Porte and A. Delaccroix, “Removal of Cd(II) and Pb(II) ions from Aqueous solutions by Adsorption onto Sawdust of Pinus Sylvestris”, J. Hazard. Mater., vol. B 105, pp. 121-142, 2003.
CrossRef
- L.A. Teles de Vasconcelos and C.G. Gonzalez Beca, “Adsorption Equilibria between Pine Bark and Several ions in Aqueous solution Cd(II), Cr(III) and Hg(II)”, Eur. Water Pollut. Control, vol. 3, no. 6, pp. 29-39, 1993.
- Vikrant Sarin, Tony Sarvinder Singh and K.K. Pant, “Thermodynamic and Breakthrough column studies for the Selective sorption of chromium from Industrial effluent on Activated eucalyptus bark”, Biores. Technol., vol. 97, pp. 1986–1993, 2006.
CrossRef
- Feng-chin Wu, Ru-Ling Tseng and Chi-Chang Hu, “Comparisions of Pore properties and Adsorption performance of KOH-activated and Steamactivated carbons”, Micropor. Mesopor. Mater., vol. 80, pp. 95-106, 2005.
- S. Karthikeyan and P. Sivakumar, “The Effect of Activating agents on the Activated carbon prepared from Feronia limonia (L.) swingle (Wood Apple) shell”, J. Environ. Nanotechnol., vol. 1, pp. 5, 2012.
CrossRef
- S. Karthikeyan and A. Baburajendran,, “Adsorption of Basic Dye (Rhodamine B) by a low cost Activated carbon prepared from Agricultural solid waste : Leucaena leucocephala Seed Shell Waste”, Nature Environ. Pollut. Technol., vol. 9, pp. 461, 2010.
- S. Karthikeyan, B. Sivakumar and N. Sivakumar, “Film and Pore Diffusion Modeling for Adsorption of Reactive Red 2 from Aqueous Solutin on to Activated Carbon Prepared from Bio-Diesel Industrial Waste”, J. Chem., vol. 7, pp. 175, 2010.
- S. Karthikeyan, K. Sakthivel and C. Kannan, “Sorption dynamics and Equilibrium uptake of basic dye (basic brown 4) using Jatroha curcas stem activated Carbon”, Rasayan J. Chem., vol. 4, pp. 519, 2011.
- A. Lawrence Justin, R. Padmavathi and S. Kalaiselvan “Study of the Physico Chemical Properties of Treated Water from Coimbatore Lake Using Ecobiosorbent”, AIP Conference Proceeding, 2020
CrossRef
- S Kalaiselvan et al. Waste Water Treatment Using Air Stripper and Natural Adsorbent, International Journal of Research and Analytical Reviews, Vol 6(1), 2019
CrossRef
- S. Mageswari, S. Kalaiselvan, P.S. Syed Shabudeen, N. Sivakumar and S. Karthikeyan, “Optimization of growth temperature of multiwalled carbon nanotubes fabricated by chemical vapour deposition and their application for arsenic removal”, International Journal of Material science, vol. 32, no. 4, pp 709-718, 2014
- M. Manjuladevi and S. Kalaiselvan “Applications of UV-Visible & FT-IR Spectral Analysis in Effluent Treatment”, Omics International, DOI: 10.4172/978-1-63278-014-0-015, 2019
CrossRef
- S. Lagergren, “ZurTheorie Der Sogennten Adsorption GelosterStoffe”, Hand linger, vol. 24, pp. 1, 1898.
- Y.S. Ho and G. Mckay, Adsorpt. Sci. Technol., vol. 18, pp. 639, 2000.
CrossRef
- W. Weber and J. Morris, “Kinetics of Adsorption of Carbon from Solution”, J. Sanit- Eng.Div. Am. Soc. Civ. Eng., vol. 89, pp. 31, 1963.
Views: 407
This work is licensed under a Creative Commons Attribution 4.0 International License.